
Precision farming
Farming is under pressure to produce more food, animal feed and fibre while using fewer chemicals. There is also a drive to use less energy and labour while at the same time improving the environmental management of land and water. One way to achieve these goals is through ‘precision farming’, the control of farm implements working the land, how they apply seeds, fertilisers and agrochemicals, and harvest the crop.
The recent Foresight report, Land Use Futures: Making the most of land in the 21st century, summed up these aspirations for the UK when it said “precision farming and technologies that monitor soil condition and water quality will enable farming to maintain high levels of productivity while reducing its environmental burden.” For this to happen, farmers have to know what is happening on their land, and the health of their crops from place to place throughout a field. Precision farming develops technologies that can locate and control the positions of farm vehicles with sensors that can analyse crops at the right scale.
Wheels within fields
🚜 A revolution in field machine design with controlled traffic farming
Controlled traffic farming (CTF) aims to confine vehicles to the smallest area on permanent traffic lanes in fields. This requires accurate and repeatable positioning, using GPS and autosteering, as well as matching machinery wheel tracks and operating widths.
The basic principle of CTF is to match the operating widths and the track centre widths (gauge) of the various vehicles used on farms. Ideally, the vehicles’ axle-track gauges, the distance between wheels on the same axle, should be as wide as possible, with all field-going equipment operating with the same gauge.
Where vehicles are not limited by the need to travel on public roads, the solution is to ‘stretch’ the tractor’s axle to match the wheel gauge of a combine harvester. CTF systems used in the UK and Europe, however, are based on tractors with typical axle-track gauges of approximately 2m, narrow enough to travel on public roads, while combine harvesters have axle-track gauges of 3m. Combine harvesters that have a wide track gauge are tolerated on public roads because they are used for only a limited period of the year. In Europe, traffic regulations force farmers to use less efficient solutions. Even with this restriction, the number of wheel tracks needed to cultivate and harvest a crop can be significantly reduced. The solution to this is to design tractors with variable axle-track gauges to vary the gauge between field and road use.
Matching operating widths so that vehicles can use the same tracks involves careful selection when purchasing, or in-house modification. Currently, a lack of commonality in machine widths makes purchasing less simple than it could be, but there are signs that the industry is addressing this issue.
This could mean a revolution in the design of field machines, with the introduction of wide-span vehicles, for track widths of, say, 10m, that can drive lengthways on the road and, by swivelling their axles, have wider tracks when on the field.
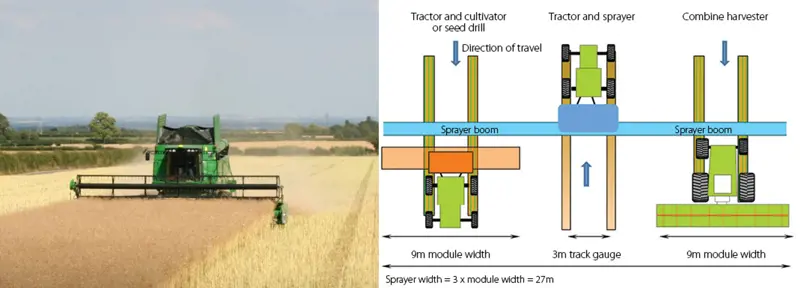
A combine harvesting the final swath in a controlled traffic farming operation. In this operation the width of the crop being harvested matches that of the header unit of the combine harvester (Left) © Institution of Agricultural Engineers assigned to Neesham Farms Ltd. Schematic of an ideal CTF system for arable crops where the tractor, implement and combine harvester track gauges are equal (at 3m) © Chamen (CTF Europe)
Accurate location
Precision farming became possible in the early 1990s, when the US declassified the global positioning system (GPS), the network of satellites developed during the cold war for military navigation. GPS, a ubiquitous technology found in most smartphones, can locate and track a vehicle’s position with an accuracy of around 10 metres. This does not provide the accuracy and repeatability required for precise field machine operations, so many farm equipment suppliers use real time kinematic (RTK) systems which employ two or more GPS receivers, connected by radio, to refine positioning accuracy. With RTK, one GPS receiver operates as a base station while the other is on the moving vehicle. RTK can provide positioning accuracy down to a couple of centimetres.
RTK can provide positioning accuracy down to a couple of centimetres
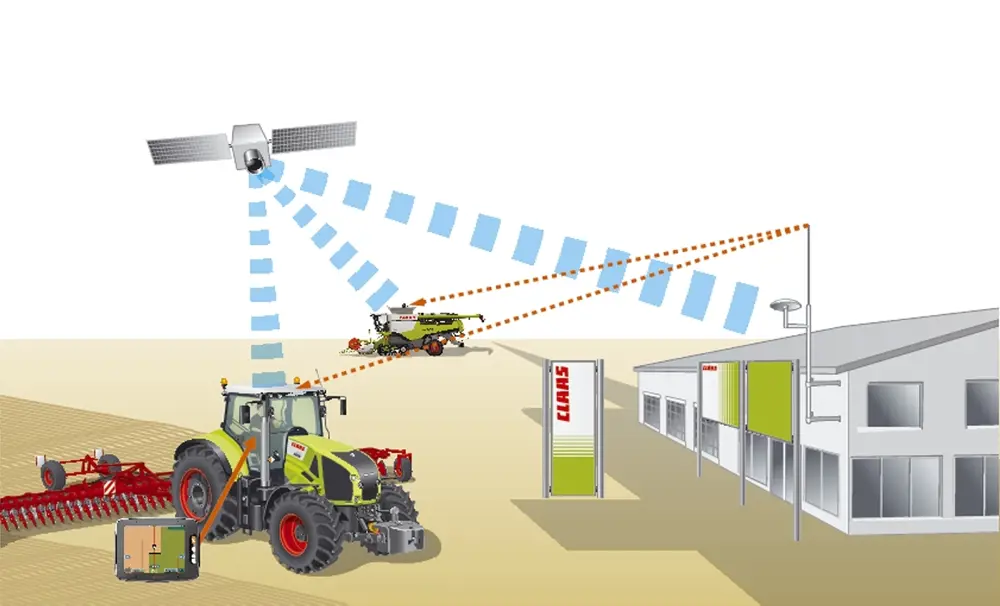
Real time kinematic (RTK) systems work by using a base station placed on a known, surveyed point that transmits correction signals via radio to dual frequency GPS equipped machines that can pick up signals from both an RTK mast and from satellites. Subject to topography, each mast provides coverage across a 20-30km radius. This system can locate the position with an accuracy of 2-3cm © Claas
In farming, one of the first sectors to utilise GPS, the development of the technology improved alongside the ability to collect data on the state of soil and crops. Knowing the location of agricultural machinery makes possible the production of yield maps by linking the position of a harvesting machine to instantaneous measurements of crop yield. Precision farming can then use these maps, along with other information, to control the application of agrochemicals, matching the distribution of fertilisers, herbicides and pesticides to the varying conditions in crops and fields.
Suppliers of tractors and agricultural machines can integrate GPS with machine guidance and automatic steering to maintain parallel pathways at the machine’s operating width across the field. Automated systems can also lift ploughs and other implements from the soil at a field’s headlands, steer a tractor into position and lower the implement in the right place for the next pass. This not only means that the equipment sticks to its intended path, but also reduces operator fatigue and increases safety: the operator can concentrate on the operation in hand rather than steering the machine.
GPS systems and automated farm machinery can also extend the working day. For example, the managers of large state farms in Heilongjiang in northeast China have reported that they achieved a 40% increase in machine productivity by allowing equipment to operate in poor light conditions and at night. As well as extending the operating period, this approach can also allow field operations to take place when the soil, crop and weather conditions are optimal, with the potential to improve crop yield and quality.
Farm vehicles that can follow an accurate and controlled route through crops can minimise the overlap between machine passes through a field, thereby avoiding the risk that some areas will receive a double dose of seeds, fertilizer and agrochemicals. By the same measure, productivity is improved by ensuring that there are no gaps where crops may not have been planted or have received the required agrochemical.
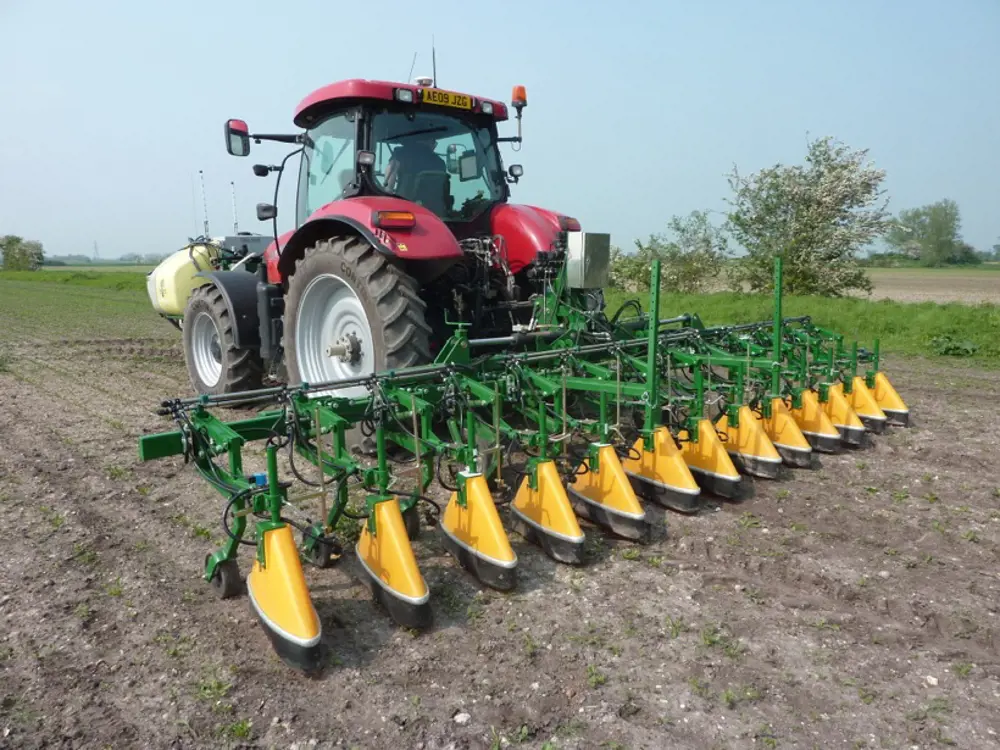
The Micron Group has developed an award-winning shielded band sprayer for cereals, roots and other narrow-planted row crops. Double-membrane shields eliminate the transfer of herbicide onto the crop, enabling drift-free weeding between rows. The tractor is operated with RTK GPS (circled) auto steer to follow the crop rows enabling the operator to concentrate on managing other operations.
Soil compaction
Tractors and harvesters have increased dramatically in size and weight since the Second World War. Tractors of 15 to 25 tonnes, more than 10 times the weight of the classic Ferguson of the immediate post-war years, are not uncommon. Fully laden combine harvesters can weigh 30 tonnes, with sugar beet harvesters weighing as much as 50 tonnes. The latter often work through the winter, when the soil is soft and wet.
Traditional vehicles can be of varying widths, so wheel traffic has the potential to damage most of a field in a single season. If not managed correctly, this traffic can compact soil, leading to significant problems in subsequent years, reducing crop yield, increasing rainfall runoff and, in some circumstances, raising the risk of flooding and soil erosion.
One way to alleviate soil compaction is through controlled traffic farming (CTF) – see Wheels within fields. CTF separates the cropped and wheeled areas, with all fieldgoing operations on the same wheel tracks. By utilising GPS and automatic steering, CTF can reduce the field area damaged by wheels from as high as 85% of the field area to 15%, and increase yields by between 9% and 35%. It can also increase infiltration of rainfall into the soil by up to 400%, reducing runoff and flooding, and save fuel by increasing the efficiency of field machinery.
It can take time for farmers to convert to CTF and to ensure that their vehicles have compatible axle widths. Despite these challenges, a growing number of farmers, accounting for more than 50,000 hectares in the UK, use CTF systems today.
Weed control
🌾 A novel approach to automate mechanical weeding
Precision farming can improve the efficiency of weeding. For example, vision guidance systems can increase the accuracy of the cultivating hoe for weed control between rows and help reduce crop damage. However, this leaves weeds growing within largely uncontrolled rows. Tillett and Hague Technology, working with the UK company, Garford Farm Machinery, and Cranfield University, developed a novel approach to automate mechanical weeding. The trick is to use a rotary disc with a segment removed to miss the crop plants and to ‘hoe’ the weeds in the crop row. The prototype machine, first demonstrated in 2007, was an almost immediate success.
Garford has produced over 80 commercial in-row ‘Robocrop’ machines for mechanical weeding in the vegetable producing areas of Australia, China and Japan and North America as well as Europe. Continued developments have added the ability to control nozzles to better-target liquids onto specific parts of individual plants, either as they are cultivated or as a separate operation. Growers can now buy larger and more sophisticated weeding machines, the largest of which includes 20 rotors.
Automated mechanical weed control is good for widely-spaced and transplanted crops such as lettuce and brassicas, particularly where the soil is dry or drying. Such approaches have limitations for onions, carrots, parsnips and leeks, which are grown with closer spacing. The use of selective herbicides in such situations is now limited because many are no longer approved by the latest regulations. A vision-guided spot-sprayer manufactured by Garford uses a similar approach to that employed in the intra-row hoe. The system uses image analysis to identify weeds within or between the crop rows. The imaging system uses a combination of factors, including plant size, colour and position. The system’s detection algorithm can target weeds and actuate spray nozzles.
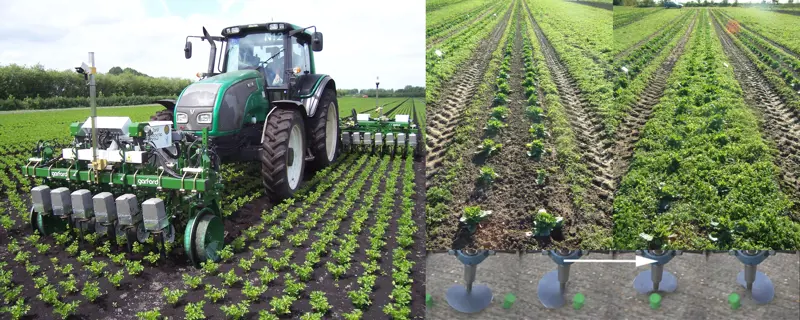
The In-Row Robocrop is working a celery crop to manually remove weeds between rows (left). Before and after images of the weed control in cabbage crop using the rotating notched disc (top right). Rotating notched disc for intra-row hoeing of wide-spaced row crops (bottom right). © Tillett and Hague Technology and Garford Farm Machinery
Mapping fields and crops
As well as the ability to control the position and operation of equipment, precision farming relies on knowing what is going on where in a field, and how crops are responding to soil conditions, pests and diseases, and the weather. Linking the GPS position of the harvesting machine to a real-time record of the mass or volumetric flow of the crop during the harvesting process provides the information required to generate yield maps.
precision farming relies on knowing what is going on where in a field, and how crops are responding to soil conditions, pests and diseases, and the weather
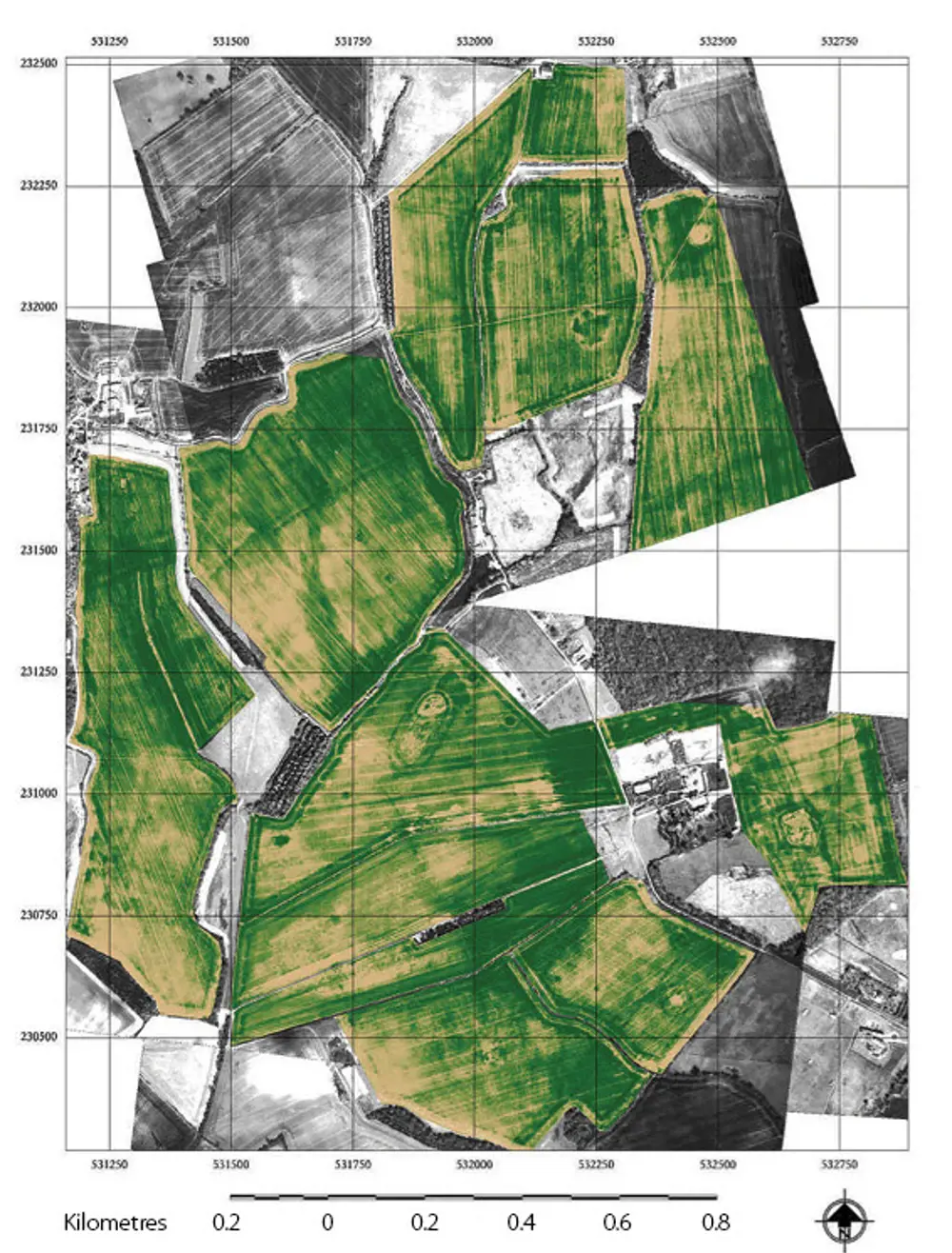
The composite aerial image shows up the differing stages of crop development within a given field using normalised difference vegetation index (NDVI) data. This can assist in determining the different rates of nitrogen fertilizer required © Cranfield University/AHDB
Variations in soil type, direction of the field slope and other factors can affect the growth and yield of a crop within a field. More critically, yield maps give farmers data on the magnitude of the differences in yield and their distribution throughout the field. Yield maps can be combined with other data, such as soil maps derived from the measurement of electrical conductivity – which senses variation in soil moisture, which in turn is related to the amount of sand, silt and clay in that part of the field. This can help in the creation of an application map to control the rate at which a fertilizer distributor, equipped with GPS applies fertilizer as it traverses the field.
The application of nitrogen is an example of how farmers have changed how they use data such as yield maps. Early management scenarios took the yield map from the previous season: if this showed an area of the field that did not perform well then farmers could apply more or less nitrogen to that area while more productive areas of the field received less, or increased amounts of, fertilizer. It soon became apparent that while planning nitrogen application maps from previous years gave a valuable insight into the variability of the field, this was not ideal. Planning how to apply nitrogen required real-time sensing of the crop’s condition.
In the UK, farmers currently measure the status of the crop canopy when, or just before, they apply nitrogen, typically at three growth stages in the development of the crop between February and May. By comparing the canopy size to that expected of a healthy crop, agronomists can produce application maps to match the spread of nitrogen to local plant conditions.
Agronomists currently use sensors on satellites, light aircraft or agricultural vehicles to map crop conditions by observing the crop’s red and near-infrared reflectance to determine the normalised difference vegetation index (NDVI). Remote sensing, though, has to contend with the weather, and the possibility that clouds will obscure the ground at crucial times.
The technology of crop monitoring has now reached a stage where farmers can buy crop monitoring as a service. For example, the precision farming company SOYL provides farmers with satellite images of crops as an aid to crop management. The company is also developing ways to control the application of agrochemicals. Pilot studies using satellite images have shown overall increases of 3% to 7% in wheat yield. Calculations of nitrogen balance based on Cranfield University’s data suggested an overall reduction in the nitrogen remaining in the soil after the harvest of approximately one third, hence improving the overall efficiency of nitrogen use and reducing nitrogen leaching.
The technology of crop monitoring has now reached a stage where farmers can buy crop monitoring as a service
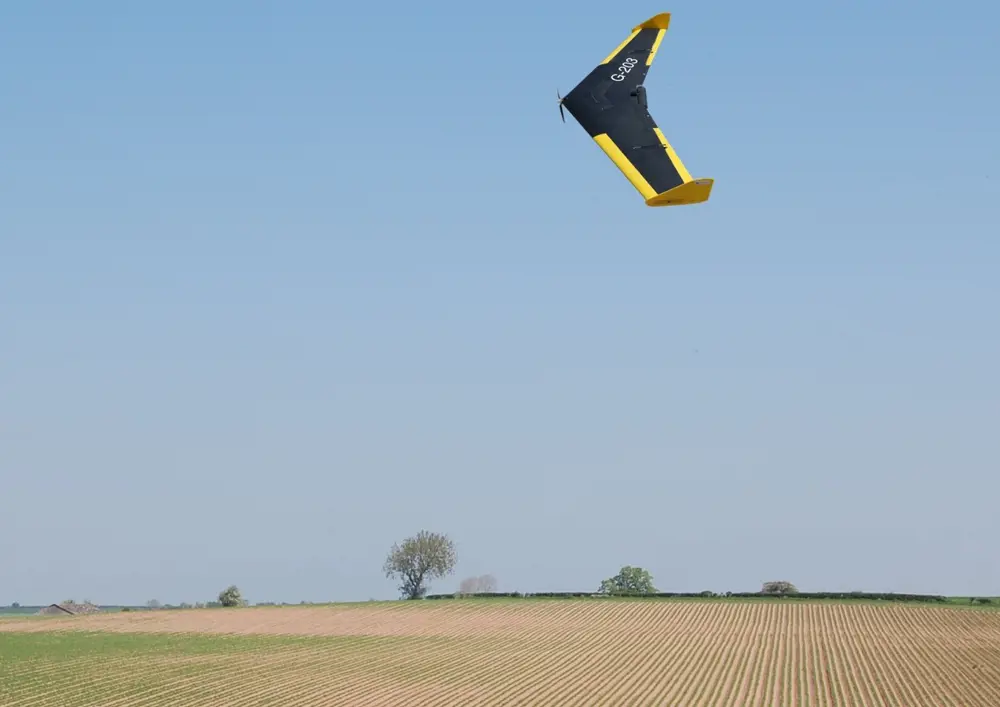
Drones today can cost anything from £10,000 for the most basic models up to £50,000 versions – so they are better suited to farmer co-operatives that can get the most use out of them. Once they can be made financially viable for broad-acre combinable crops, farmers will be able to make full use of the information provided by these surveillance systems to cut input costs or improve yields © URSULA Agriculture 2015
More recently, small-unmanned aerial vehicles (UAVs), popularly known as drones, have gathered aerial data. UAVs can fly below clouds, quickly scanning fields and immediately transmitting the data. The challenge is to make sensors light enough so that small UAVs can cover a sufficiently large area in a single flight and carry the camera payload.
The company URSULA Agriculture uses UAVs to image crops. It can then provide growers with information on weed problems and can forecast yields for crops such as lettuce and onions. The company is also investigating disease mapping in potato crops and is trying to identify areas of persistent blackgrass in cereal crops. The objective is to deliver pesticides to their targets more effectively and to reduce the use of chemicals. URSULA Agriculture’s UAV data can also help farmers when they make decisions on which varieties to grow, as well as their choice of which machinery to use and rotation planning.
The ultimate advancement for crop analysis is to place sensors on tractors. These measurements are less prone to problems caused by adverse weather and provide the closest and most accurate real-time assessments of a crop’s state. However, unless data collection and nitrogen application can happen simultaneously, tractor-mounted sensors require additional, relatively slow field operations to gather data on the state of the crop. The fertiliser company Yara provides a commercial service using on-board algorithms to estimate the required application rate for fertiliser. The algorithm is programmed from the results of many field studies, and recent versions now permit the addition of local field knowledge that the farmer or the agronomist may wish to take into consideration.
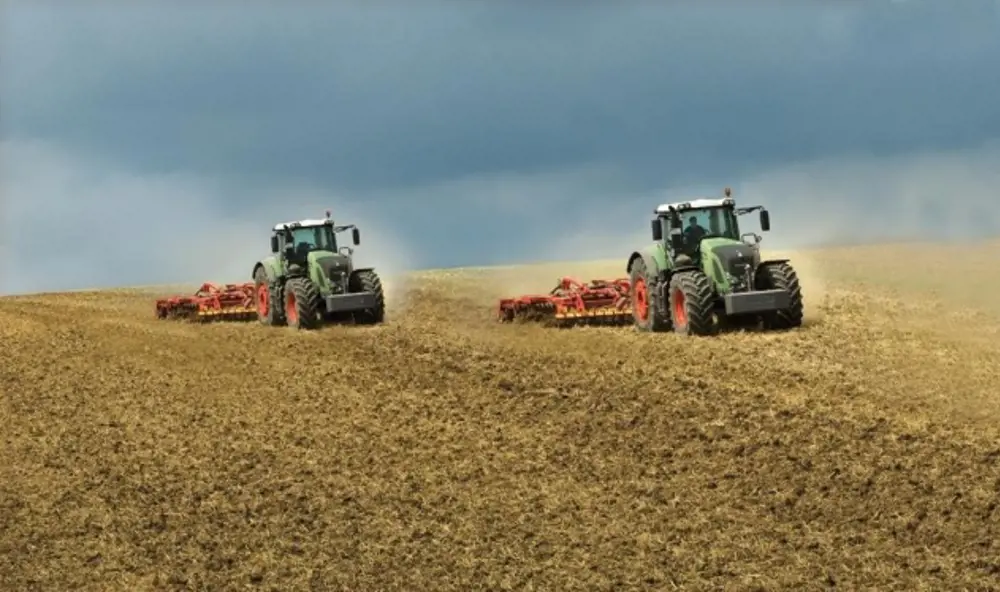
In Germany, tractor maker Fendt has already developed a way for farmers to control two tractors at the same time. In this system, the driver is based in the lead tractor while another fully automated machine follows behind, taking its operational directions through GPS signals and radio connections © Fendt
Herbicides
As well as knowing when and where to apply fertilisers, farming has to face the challenge of weed control and pressure to reduce the use of herbicides. Growers of field vegetable and salad crops, for example, try to minimise the use of herbicides for weed control, partly in response to customer pressure, but also because of crop resistance and environmental issues, such as groundwater contamination. Quality salad and leafy vegetable products do require high levels of weed control, however, so reducing the use of chemicals increases the need for other methods – see Weed control.
Precision farming can also help agriculture to adapt to the shrinking armoury of herbicides that farmers are allowed to use. Over the past decade, their introduction has been limited, due to the increasingly stringent regulatory requirements, the cost of development and the size of markets. As a result, controlling grass weeds in cereal crops is now a major issue for arable farmers. The weeds are resistant to the selective herbicides that have been the mainstay of weed control in arable crop rotations.
One technological approach to maintaining an acceptable level of weed control involves growing crops with a wider space between rows, typically 20 to 25cm rather than the traditional 10 to 24cm, and using precision guidance to deliver a non-selective herbicide to the area between rows. Machinery makers have developed commercial systems that use either computer vision to detect crop rows, and the gap between them, or RTK GPS to enable units to be positioned with accuracies in the order of 25mm. Here the technique used is to deploy specialised nozzle systems, shrouds and shields to protect crops from spray drift.
An underlying theme of these developments in precision farming is the need to know and control the position of the vehicles that are applying agrochemicals and removing weeds
An underlying theme of these developments in precision farming is the need to know and control the position of the vehicles that are applying agrochemicals and removing weeds. Vehicles that can operate free of the controlling hand of the driver are already making inroads into agriculture. As with automobiles, we can expect to see driverless tractors in the not-too-distant future. These will use GPS, machine guidance and auto-steer technologies. These may eventually develop into fully autonomous vehicles.
However, farm vehicles can be very much larger and heavier than cars. The major concern for fully autonomous 15-tonne, 300hp tractors is the issue of liability should the control and fail-safe shutdown systems malfunction. A partial solution is the adoption of a ‘master and slave’ system, where one or more machines follow an operator-controlled lead machine. In the UK, smaller robotic field machines will probably find an initial application in crop protection, weed control and harvesting in horticultural and field vegetable production.
Next steps
Through developments like those described here, agricultural engineers can play an important part in feeding people more efficiently and with less damage to the environment. Engineering solutions such as those underlying precision farming systems can buy time until agriculture can reap the benefits of longer-term research into plant and animal genetics. Bettering the precision and efficiency in farming operations has already helped to increase crop yields, and to reduce inputs and labour. It has also brought environmental benefits by reducing residual nitrogen, and by reducing or replacing herbicides with spot and mechanical weed control. It has also improved the infiltration of rainfall into the soil, potentially reducing flooding risk and increasing the economic viability of agriculture.
We can expect to see further advances in agricultural engineering, with, for example, the rise of robotics. However, for this to happen, we need to understand the role that engineering can play in agriculture. As the Institution of Agricultural Engineers (IAgrE) said in its report Agricultural Engineering: a key discipline enabling agriculture to deliver global food security, “The contribution of engineering needs to be more widely recognised in meeting societal challenges in global food security and contributing to economic growth, and an engineering community addressing these issues needs to be established with effective links to the key stakeholders.”
The contribution of engineering needs to be more widely recognised in meeting societal challenges in global food security and contributing to economic growth
the Institution of Agricultural Engineers (IAgrE)
The example of precision agriculture demonstrates the assertion in the IAgrE’s report that “Sophisticated sensing technologies like real-time computer-based image analysis can be used with confidence to control agricultural machines, and permit, for example, effective weed management with minimal pesticide.” With its long-standing and globally recognised tradition in agricultural engineering, the UK is well placed to lead the way.
The next few decades of population growth will place huge strains on the way we need to farm. Agricultural machinery, along with its associated products and services, will be key to providing new technology platforms for improved field management. To facilitate this, there will need to be wise leadership, sufficient investment and well-trained operators, as well as engineers and researchers in the field. In short, we need to protect our agricultural capital and develop our human capital.
***
The author would like to thank the following for their contributions to this article: Dr Tim Chamen (Controlled Traffic Europe), Dr Paul Miller (NIAB-TAG), Dr Nick Tillet (Tillet and Hague Technology Ltd), Professor Simon Blackmore (Harper Adams University) and Michael Kenward OBE.
This article has been adapted from "Precision farming", which originally appeared in the print edition of Ingenia 64 (September 2015).
Contributors
Professor Richard Godwin FREng is an Honorary Fellow of both the Institution of Agricultural Engineers and the Royal Agricultural Society of England, and a Fellow of the American Society of Agricultural and Biological Engineers. He is Emeritus Professor of Agricultural Engineering at Cranfield University and Visiting Professor at Harper Adams University.
Keep up-to-date with Ingenia for free
SubscribeRelated content
Food & agriculture
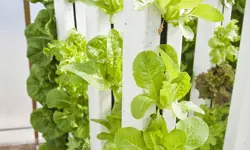
Farming straight up
Hydroponics, growing plants without soil in nutrient-enriched water, is a technique that has been used in some form for centuries. As the global population grows and food security is threatened, its faster growth and larger yields have become increasingly important.
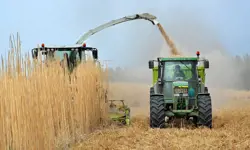
What role for biofuels in low-carbon UK transport?
Biofuels have a role to play in meeting the UK’s climate change commitments. Sustainable Chemical Engineering Professor Adisa Azapagic FREng sets out why biofuels made from wastes and by-products in different sectors are particularly important to these efforts.
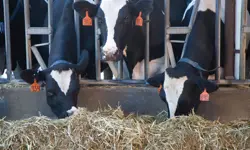
The automation of dairy farms
Robotic milking is not a new innovation, but the systems are increasingly being adopted by UK dairy farms. Science writer and broadcaster Geoff Watts learned from John Baines, Technical Director at Fullwood, how these systems are being engineered to do more than just milk cows.
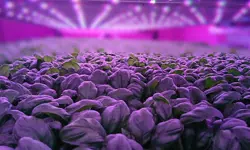
Vertical farming for future growth
More of us live in cities than ever before and the global population is estimated to reach 11.2 billion by 2100, creating mounting challenges for agriculture. Growth Solutions grow produce under lights in vertically stacked towers to help meet growing demand for food.
Other content from Ingenia
Quick read
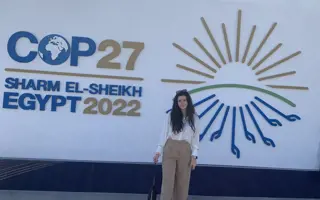
- Environment & sustainability
- Opinion
A young engineer’s perspective on the good, the bad and the ugly of COP27
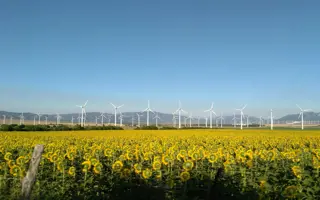
- Environment & sustainability
- Issue 95
How do we pay for net zero technologies?
Quick read
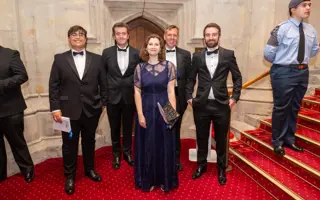
- Transport
- Mechanical
- How I got here
Electrifying trains and STEMAZING outreach
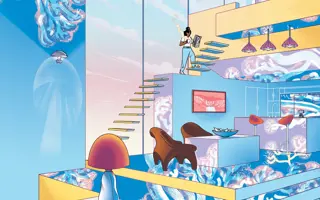
- Civil & structural
- Environment & sustainability
- Issue 95