
Why 1960s CCD technology is at the frontier of space exploration
Did you know?
All about charged coupled devices (CCDs)
- CCDs have been used in astronomy and planetary science for decades
- CCDs are at the heart of scientific instruments onboard NASA’s Perseverance rover
- These instruments are discovering if rocks on Mars show evidence of past or present living organisms on the planet
- The CCD sensors on Perseverance are designed to withstand the intense cosmic radiation present in space and on the surface of Mars
The CCD, or charged coupled device, is a type of microchip that can turn photons, the elementary particles of light, into electronic charge, then read the resulting signal pixel by pixel and translate it into visual images or light spectra.
Invented in the late 1960s by Americans Willard Boyle and George E. Smith (who were awarded the Nobel Prize for Physics for the invention in 2009, with Smith also receiving the Queen Elizabeth Prize for Engineering Innovation in 2018 – see ‘Image revolutionaries’, Ingenia 73), CCD technology ushered in the era of digital imaging and photography. Although consumer cameras and camcorders eventually switched to cheaper mass-manufactured CMOS (complementary metal-oxide-semiconductor) sensors, demanding scientific imagers still rely on CCDs.
In astronomy and planetary science, CCDs have been a mainstay for decades. CCD sensors enable the famous Hubble Space Telescope to take breathtaking images of distant galaxies. CCD sensors were on both the comet-chasing Rosetta spacecraft as well as the lander Philae. They sat inside the instruments of NASA’s Mars explorers Curiosity, Spirit and Opportunity. They enabled the Kepler Space Telescope to detect the minuscule dips in brightness of distant stars caused by transits of orbiting exoplanets. CCD technology inside the cameras of NASA’s New Horizon spacecraft provided humans with the first detailed look at Pluto, the most distant large body in the Solar System.
Nearly every mission that has carried cameras and various kinds of imaging instruments in the past three decades has had CCD sensors on board, and many of these sensors have been manufactured by UK-based engineering firm Teledyne e2v.
“For space applications, the number one criterion when choosing a technology is whether someone has flown it already and proved it reliable,” says Paul Jerram, Chief Engineer at Teledyne e2v. “Space agencies are very conservative and often prefer technology that is many years old.”
When NASA decided in 2012 to develop a new Mars rover, with the grand goal to search for traces of life on Mars, the agency’s engineers again turned to Teledyne e2v to provide its tried and tested CCDs.
Nearly every mission that has carried cameras and various kinds of imaging instruments in the past three decades has had CCD sensors on board, and many of these sensors have been manufactured by UK-based engineering firm Teledyne e2v.
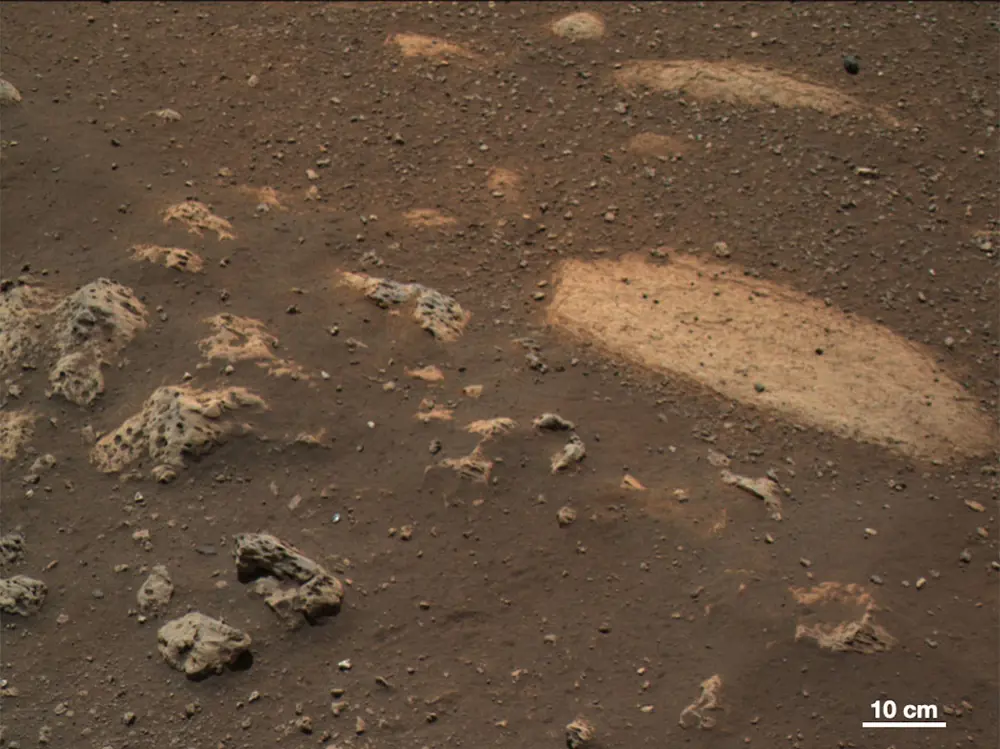
Taken in February 2021, this image from the Mastcam-Z instrument on NASA’s Perseverance rover shows the first target for analysis by the rover’s SuperCam instrument © NASA
SHERLOC and SuperCam
SHERLOC and SuperCam are two of the seven scientific instruments on board of the Perseverance rover. Both instruments are complex, consisting of optical cameras and analytical devices called spectrometers. These spectrometers detect light reflected by materials on the surface of the Red Planet and split it into to the wavelengths of the electromagnetic spectrum. Because different chemical materials absorb and reflect light differently, these spectral signatures then reveal to the scientists the chemical composition of whatever they are looking at. In the case of SHERLOC and SuperCam, the goal is to find out whether rocks on the Martian surface contain molecules suggesting past or present existence of living organisms on the planet.
“Our CCDs sit at the heart of these spectrometers,” says Jerram. “As the light enters the detector, it goes through a diffraction grating that splits the spectrum across a range of wavelengths before it falls onto the CCD. Then you detect the signal across this wide wavelength range."
The adventures of NASA's Perseverance rover
Just roving around on Mars, searching for traces of water...
Since 8 February 2021, NASA’s Mars Perseverance rover has been traversing Jezero, a 50-kilometre-deep crater created by the impact of an ancient meteorite. Scientists believe that Jezero, which means ‘a lake’ in several eastern European languages, was once flooded with water. This past presence of water is what makes Jezero an intriguing target for a mission, the goal of which is to search for signs of past life and potentially habitable conditions. About the size of a small car, Perseverance is equipped with seven scientific instruments.
The Perseverance mission is the first step in a chain that will ultimately bring a sample of Martian soil to the Earth at some point in the early 2030s. Equipped with a small drill, Perseverance will scoop dirt from Jezero and prepare over 30 sealed samples for a later mission to collect. To ensure the samples have the highest scientific value, and the best probability to harbour traces of past life, the rover uses a suite of sophisticated instruments that analyse the chemical composition of rocks and look for organic compounds. Perseverance also carries a device that makes oxygen from carbon dioxide in the Martian atmosphere, a key technology for future human exploration, and another tool that analyses weather in the crater.
Since sending a rover to Mars costs a lot of money, the engineers want to squeeze as much information out of the instruments as technically possible. Teledyne’s CCD42-10 product used in SHERLOC and SuperCam was designed to be sensitive to a range of wavelengths that exceeds that of the human eye – it includes the visible wavelengths of 400 to 700 nanometres, which the human eye can detect, as well as the near-infrared wavelengths of 700 to 1,000 nanometres. The devices turn light into a valuable scientific signal with nearly 100% efficiency, meaning they turn nearly every single photon that hits the device into an information-generating electron.
“We make the CCDs sensitive down to the ultraviolet and up into the near-infrared, from under 200 nanometres up to 1,100 nanometres,” Jerram says. “Then you get a spectrum out of the instrument and you can see the various peaks and troughs that represent the various parts of the spectrum. That tells you about the presence of certain minerals and materials, especially organic materials."
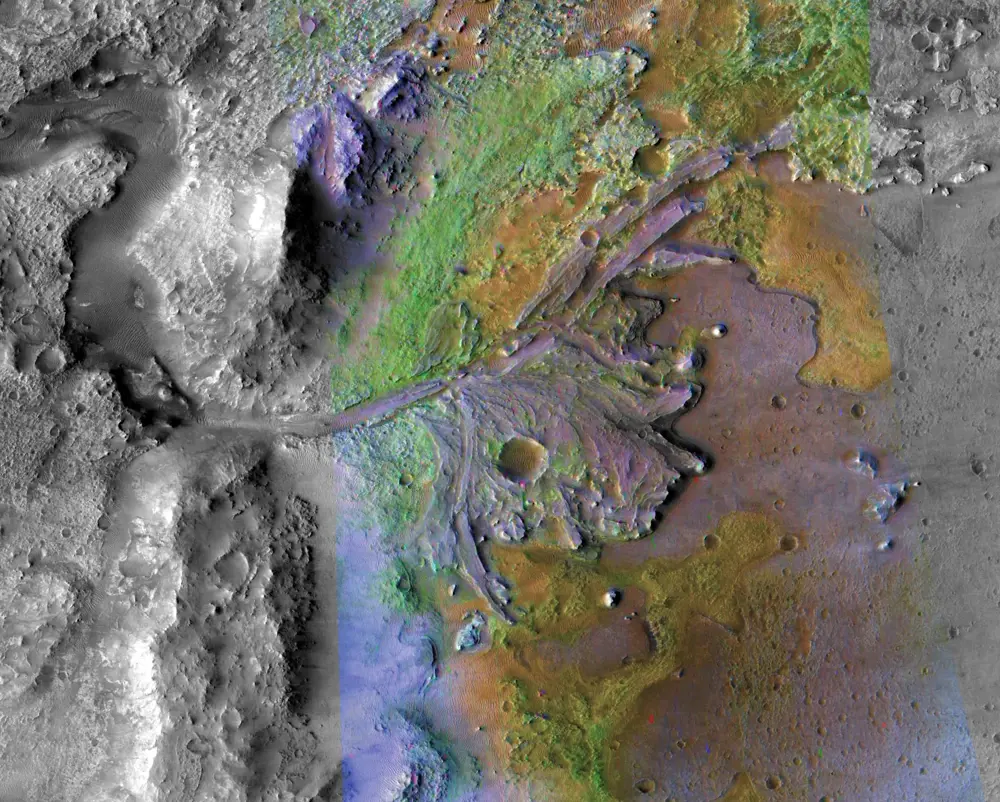
This image of Jezero Crater, the landing site for the Mars Perseverance rover, was taken by instruments on NASA’s Mars Reconnaissance Orbiter © NASA
What is a CCD?
Key to scientific imaging
The CCD, or a charged coupled device, is a type of integrated circuit, a complex electronic circuit etched on a single small piece of silicon. The CCD is made of light-sensitive elements, called pixels. As photons hit the chip’s surface, they generate electronic charge in the pixels. The number of electrons generated in the pixel after photon impact is directly proportionate to the intensity of the light on the pixel in the resulting image. By measuring the number of electrons in each pixel, the device reconstructs the scene in front of the camera.
The CCDs inside scientific instruments are designed to maximise what scientists can learn from the mission. In the case of CCDs in space telescopes and Mars rovers, the key is to capture every photon that hits the detector and turn it into an electron, a factor called the quantum efficiency.
Engineers can increase the quantum efficiency of CCDs by controlling how light illuminates the chip. If a chip is illuminated from the back, the light doesn’t have to pass through the layer of polycrystalline silicon that usually covers the front of the chip, which might reduce the number of photons that pass through. To enable a chip to be used this way, however, requires sophisticated engineering techniques and increases the cost of the chip.
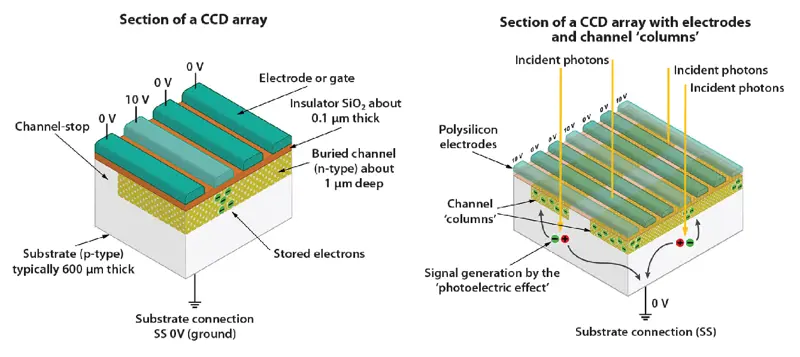
Charge collection within a CCD image sensor © Teledyne e2v
Indestructible sensors
Before setting off for its journey to Mars, the 2,000 by 500-pixel CCDs had to undergo a battery of tests designed to prove that the sensors would survive the shocks and vibrations of the rocket launch and Martian landing. They also needed to withstand the 100°C thermal fluctuations during the cruise to Mars as well as on the planet’s surface, and the exposure to cosmic radiation, which is much stronger on Mars than on the Earth. The engineers also had to make sure that the sensors would perform flawlessly for at least for the planned mission lifetime of around 24 months despite the punishing conditions.
“When you produce a sensor for Earth, you make one and you deliver it,” says Jerram. “But if we want one sensor to go into an instrument like SHERLOC, we might make something like 30 or 40 sensors and deliver two or three, the flight model and the spares. We would test the rest up to destruction.”
The shocks and vibrations induced during the tests would be more violent than those expected during the journey, the thermal swings would be greater and the temperature would change faster. Failures in space are costly: it took a repair mission worth hundreds of millions of dollars to fix the short-sighted Hubble Space Telescope in the 1990s. But Hubble orbits merely 550 kilometres above the Earth. Mars is, at its closest, more than 50 million kilometres away, beyond the reach of any currently feasible human space mission.
The sensors inside Perseverance are essentially the same as those used in instruments on the earlier rovers Curiosity, Spirit and Opportunity, so much of the challenging engineering work had already been done ahead of the earlier missions. In addition to being tried and tested, the advantage of the CCD technology compared to CMOS, Jerram says, is the relative simplicity of its design, which makes it “inherently suitable” for space applications.
“A CCD is actually quite simple in principle and typically has something like two transistors on it and it just moves charge about, detects the charge and converts it to an output signal,” Jerram says. “There is much more to consider with CMOS sensors.”
The CCD sensors on Perseverance are designed to withstand the intense cosmic radiation present in space and on the surface of Mars, which unlike the Earth has no protective magnetic field.
The CCD sensors on Perseverance are designed to withstand the intense cosmic radiation present in space and on the surface of Mars, which unlike the Earth has no protective magnetic field.
What's on board the Perseverance rover?
👽 The suite of next-generation technologies that aim to detect possible signs of life on Mars
Mastcam-Z:
A set of two cameras located on the rover’s mast that capture panoramic and stereoscopic images of the rover’s surroundings. The instrument assists with rover operations and can determine the mineralogical composition of Jezero crater.
MEDA (Mars Environmental Dynamics Analyzer):
A set of sensors that provide measurements of temperature, wind speed and direction, pressure, and relative humidity in Jezero crater, and analyse the size and shape of dust particles in the atmosphere.
MOXIE (Mars Oxygen ISRU Experiment):
A technological demonstrator designed to show how future Martian explorers would manufacture oxygen for breathing from carbon dioxide in the atmosphere of Mars.
PIXL (Planetary Instrument for X-ray Lithochemistry):
An X-ray fluorescence spectrometer combined with a high-resolution imager that can analyse fine elemental composition of materials on the surface of Mars with unprecedented detail.
RIMFAX (Radar Imager for Mars’ Subsurface Experiment):
A ground-penetrating radar measuring the geological structures in the subsurface of Jezero crater with 1 centimetre resolution. RIMFAX can detect water, ice or salty brines up to 10 metres under the surface of Mars. Water-rich environments hold the highest promise of harbouring traces of past or present life.
SHERLOC (Scanning Habitable Environments with Raman & Luminescence for Organics and Chemicals):
A spectrometer that will provide fine-scale imaging and uses an ultraviolet (UV) laser to determine fine-scale mineralogy and detect organic compounds. SHERLOC is the first UV Raman spectrometer to fly to the surface of Mars and will provide complementary measurements with other instruments in the payload.
SuperCam:
An instrument that takes images of rocks in the Jezero crater and analyses their chemical composition. The instruments can detect organic compounds in the rock from a distance without having to interact with them.
Protecting CCDs from the harsh realities of space flight
It’s not only the sensor itself that has to be robust. A CCD destined for space needs carefully engineered packaging that will not only protect it against the turbulences of space flight but also prevent cracks as both the packaging and the sensor expand and contract in the swinging temperatures.
“When we design a package for a CCD that will go to space, we have to do a lot of thermal modelling,” Jerram says. “You have to make sure that the material has a good thermal expansion match to the silicon substrate of the CCD.”
The packages protecting the CCDs in SHERLOC and SuperCam are made of the ceramic materials aluminium nitride and aluminium oxide. These can withstand temperatures of up to 150°C.
Keeping earth-germs out
Teledyne e2v first developed this type of CCD sensor for an Earth-based telescope operated by the Royal Observatory in Greenwich. Since then, the technology has been included on 49 space missions (the number is still growing) and has been used in countless ground-based instruments.
Yet, making a chip for Mars does come with specific challenges. The mission of a life-detecting rover might be completely ruined if the vehicle brought living organisms with it from the Earth. Astrobiologists are concerned about the possible contamination of Mars with Earth’s bugs and the threat they might pose to the Martian natives.
Unlike most of the Mars-bound equipment, chips don’t need to be sterilised by chemicals or heat. Still, strict regulations apply to them as to how many bacterial spores and dust particles they are allowed to contain.
“Contamination is an important issue,” says Jerram. “All the fabrication, packaging and testing is done in highly controlled clean rooms. The contamination that you are allowed is extremely low, so we have to keep the devices clean all the way through.”
SHERLOC and SuperCam will ultimately help scientists select pieces of rock that the rover will then extract and place into small tubes. These tubes will be stored on the Martian surface and wait for a Sample Return Mission to collect them and bring them to the Earth at some point in the early 2030s. The UK-made technology will help answer the big question of whether life exists or has ever existed on Mars.
***
This article has been adapted from "At the frontier of space exploration", which originally appeared in the print edition of Ingenia 88 (September 2021).
Contributors
Tereza Pultarova
Author
Paul Jerram has worked in Teledyne e2v’s imaging division for nearly 25 years, after roles in different technology areas at the company. As Chief Engineer for Space Imaging, he ensures that Teledyne e2v’s imaging technology is developed to meet customers’ needs. Paul has a PhD in atomic physics from University College London.
Keep up-to-date with Ingenia for free
SubscribeRelated content
Aerospace
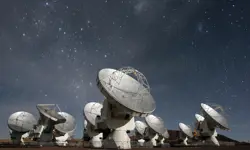
ALMA – the high altitude observatory
The Atacama Large Millimetre/submillimetre Array (ALMA) is the largest and most expensive ground-based telescope built, revolutionising our understanding of stars and planetary systems. Building it in the Atacama Desert in Chile required the ingenuity of hundreds of engineers.
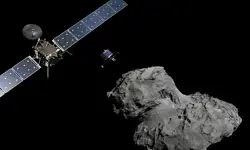
Communicating with outer space
The Royal Academy of Engineering awarded a team at BAE Systems the Major Project Award in June 2016 for their development of a powerful satellite modem system, pivotal in enabling the precise control of the pioneering Rosetta spacecraft and the first-ever soft landing of a spacecraft on a comet.
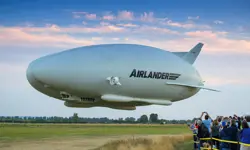
An aircraft like no other
The Airlander made headlines when it embarked on its first test flight in August 2016 as the world’s largest aircraft. Chris Daniels at Hybrid Air Vehicles Limited, and David Burns, Airlander’s Chief Test Pilot, talk about the engineering that helped it reach this stage and plans for the craft’s future.

Q&A: Lucy Harden
Lucy Harden is a mechanical engineer on BAE Systems’ Digital Light Engine Head-Up Display development programme. She devises innovative solutions for pilots to display essential flight information that sits directly in their line of sight and is overlaid onto the real world.
Other content from Ingenia
Quick read
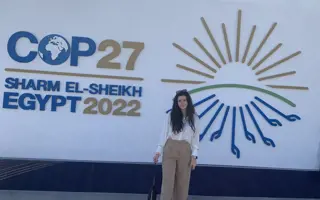
- Environment & sustainability
- Opinion
A young engineer’s perspective on the good, the bad and the ugly of COP27
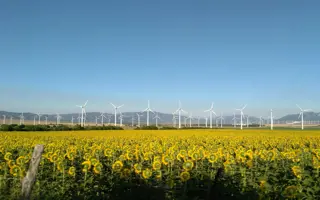
- Environment & sustainability
- Issue 95
How do we pay for net zero technologies?
Quick read
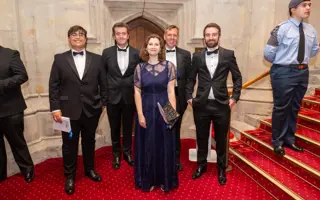
- Transport
- Mechanical
- How I got here
Electrifying trains and STEMAZING outreach
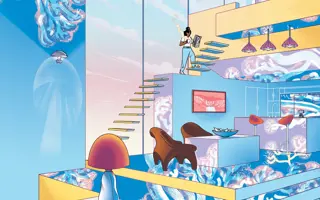
- Civil & structural
- Environment & sustainability
- Issue 95