
The smart sensors enhancing safety
💬 Shini says...
Ingenia 100 guest editor Dr Shini Somara tells us her thoughts
Have you ever thought about washing up liquid becoming contaminated during its manufacturing process? I hadn’t. I was therefore fascinated to read this insightful article. Ideas, creativity and lateral thinking are always sparked when you learn about something new. And it is often the more inconspicuous problems that offer the most intricate of engineering challenges to solve. And what is really exciting is that solutions can be transferred. This article talks about biosensor technology in a branch of chemical engineering, but who knows where else these innovations could lead!
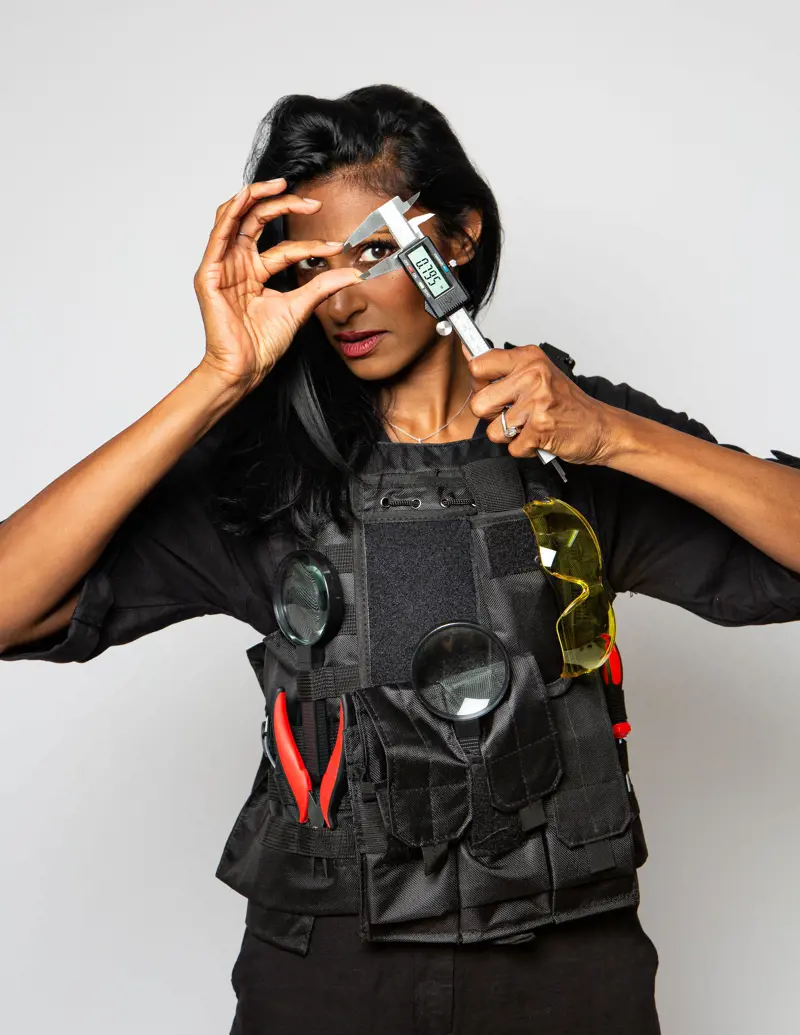
© Dr Shini Somara
From the moment that chemicals arrive at factories until an end product is bottled, those on the manufacturing line must diligently follow rigorous cleaning and sanitation procedures on a regular basis to eliminate any chance of microbial contamination. This can lead to undesirable changes in the product, which may lead to wastage and product recalls, as well as losing the trust of consumers. Therefore, manufacturers carry out microbial analysis at every step of the manufacturing process, from the arrival of chemicals to the bottling of the product. This is a vital but time-consuming and resource-intensive process.
As part of the digitisation of its supply chain, global consumer goods company P&G (Procter & Gamble) is using advanced technologies and the Internet of Things (IoT) to make its manufacturing more efficient. As the producer of household names such as Ariel, Oral B, Head & Shoulders, and Fairy, products must meet strict safety regulations and quality checks.
P&G’s processes involve regular cleaning and thermal sanitisation of the pipework. Establishing a truly clean state before sanitisation is challenging without the aid of monitoring devices. The company’s testing for microbial contamination is typically carried out ‘off-line’ or ‘at-line’, where samples are analysed away from the production line – in a separate lab for example – in a process that can take up to three days. The company recognised an opportunity to enhance these procedures in the production of its Fairy washing up liquid in a London-based factory. It actively sought a sensor technology that could be used ‘in-line’ to directly monitor the inside of the pipes for microbial contamination in real time. By implementing such sensors, P&G technicians aimed to gain accurate information on the product and pipe conditions, focusing the need for external testing. This would allow them to make informed decisions on the cleaning intervals within the factory, reducing manual effort.
Industry and manufacturing 4.0
How digital transformation is reshaping the manufacturing industry...
The integration of innovative engineering and advanced technologies into the supply chain is known as Industry 4.0. This has led to the emergence of ‘smart’ factories that use digital monitoring, optimisation and automation in every step of the manufacturing process to boost productivity.
For example, predictive maintenance is one such approach. This uses sensors and data analytics to create digital twins – virtual models of physical processes – that can predict equipment failures before they occur and therefore avoid delays in production. Another example can be seen in the use of the Internet of Things (IoT), which connects machines and devices across the factory floor, for seamless data exchange.
Manufacturing processes also use artificial intelligence and machine learning to optimise supply chain management, improving aspects such as inventory control. Adopting these digital technologies can help companies enhance their operations, making it easier for them to produce products faster and more consistently. The technologies can save time by quickly detecting problems, enabling manufacturers to make quicker decisions during production based on accurate real-time information. Digital systems also make it easier for them to control process flow, know stock levels and perform quality control.
Sensing in industrial environments
Sensors and instrumentation play a central role in the transition to Industry 4.0, with increasing use across many industries, including food, agriculture, chemical, and defence, to collect real-time data for monitoring and controlling processes. For example, the data collected in processes such as temperature regulation, pressure control, chemical composition, or bacterial growth can inform quality control, predict maintenance needs, and improve processes. Better control of these processes is also crucial for waste management.
The sensor P&G required had to be reliable, contactless and ultra-sensitive. It also had to be durable enough to withstand the chemicals and pressures in industrial pipes over a long period, as well as low cost for large-scale implementation to be economically feasible.
Many sensors in development are sensitive enough to detect small bacterial fluctuations, but this increased sensitivity makes them more vulnerable to external vibrations and temperature changes, which can interfere with microbial measurements. Vibrations can cause tiny displacements, or misalign optical or electronic components, within the sensor, leading to incorrect readings. This is a significant challenge in industrial settings where acoustic vibrations from pumps, fans and mechanical movements from seismic activity are unavoidable. For sensors that are particularly sensitive, even minute vibrations can introduce noise into readings and affect results. Slight changes in temperature can change a sensor’s sensitivity, as sensors are often calibrated to work at a specific temperature. Commercially available sensors that can withstand these environments are generally much less sensitive. After an extensive market search, the P&G team could not find a suitable device and realised that innovative engineering and advanced technology were needed.
What is optical sensing?
A sensor is a device that detects and responds to changes in the physical environment, converting these changes into electrical signals that can be processed to measure different conditions. Optical sensors use light to make these measurements. They are highly sensitive, and since they use light, they don’t need to touch the sample, which is ideal for not interfering with the sample environment.
Examples of optical sensors used to monitor manufacturing processes include Raman spectroscopy systems, which detect small frequency changes in light scatter from an object, and infrared sensors, such as those used in pharmaceutical manufacturing to monitor chemical reactions and composition. These sensors are precise and can detect minuscule changes, but they can be expensive and require specialised knowledge to operate.
Another type of optical sensor that is just beginning to be used in process control is the refractive index sensor. It measures changes in refractive index – which is how light travels through a substance – to detect changes in the environment. When the composition of a liquid changes, its refractive index does too. An optical sensor uses a light source to detect changes in the refractive index and convert them into useful data.
Turning to UK research
During the technology scouting process, P&G’s external Connect and Develop team became aware of work being carried out by a multidisciplinary team based at the University of York that specialised in innovative sensor technologies, led by Professors Thomas Krauss and Steve Johnson. P&G approached them to adapt their work for a pilot project funded by Innovate UK, and the team eagerly accepted the challenge.
Through the project, the team discovered that some of the sensor technology they had been working on, originally developed for biomedical disease diagnostics, could potentially be repurposed to detect the bacterial biofilms that P&G wanted to target. This technology, a patented optical-sensing device called the chirped guided-mode resonance sensor (chirped-GMR) (see ‘Guided-mode resonance explained’), showed promise in early experiments. The next step was adapting it for industrial use and changing its design.
🔦 Guided-mode resonance explained
Guided-mode resonance occurs in nanostructured thin films made with layers that can guide light and grating elements that diffract it. Shine a flashlight through a series of evenly spaced slits, and the light will interfere to create bright and dark spots. Reduce the spacing of the slits to the size of the wavelength of light, and the diffraction will direct the light into the grating layer. Moreover, the grating then also acts as a distributed reflector, which sets up a surface resonance. This is when light hits a material and the energy gets trapped on its surface, causing its particles to vibrate in sync. This then creates a strong localised wave of energy that can be seen as a signature sharp peak in the reflected spectrum.
As the surface resonance is formed from multiple reflections that interfere constructively, it is highly wavelength dependent. This is why its signature is a sharp peak in its reflected spectrum. This peak shifts when there is a change in the environment’s refractive index, which is how these structures can detect environmental changes.
The research team varied the spacing of the grating elements along the length of the sensor. This is comparable to having a row of slits that gradually get closer together or further apart. This ‘chirped’ design – meaning that the frequency gradually increases or decreases, similar to a bird’s chirp – allowed them to represent the spectral signature of the resonance as imaging data. For example, a change in spectrum becomes a change in position on the sensor.
Such a change can be readily imaged with a simple low-cost CMOS (complementary metal-oxide semiconductor) camera. In this way, the chirped GMR sensor combines the sensing and the spectrometer function in a single element.
To address the issue of mechanical and thermal noise that could interfere with industry measurements, the researchers changed this grating structure further into a bowtie design. The mirror symmetry of the bowtie helped to cancel out noise from physical disturbances by moving both resonances in opposite directions. When a physical disturbance, such as vibrations, occurs it typically affects both sides of the bowtie equally but in opposite directions, leading to cancellation of unwanted noise.
This precise engineering ensured that the signal produced from the sensor was only caused by the change in refractive index.
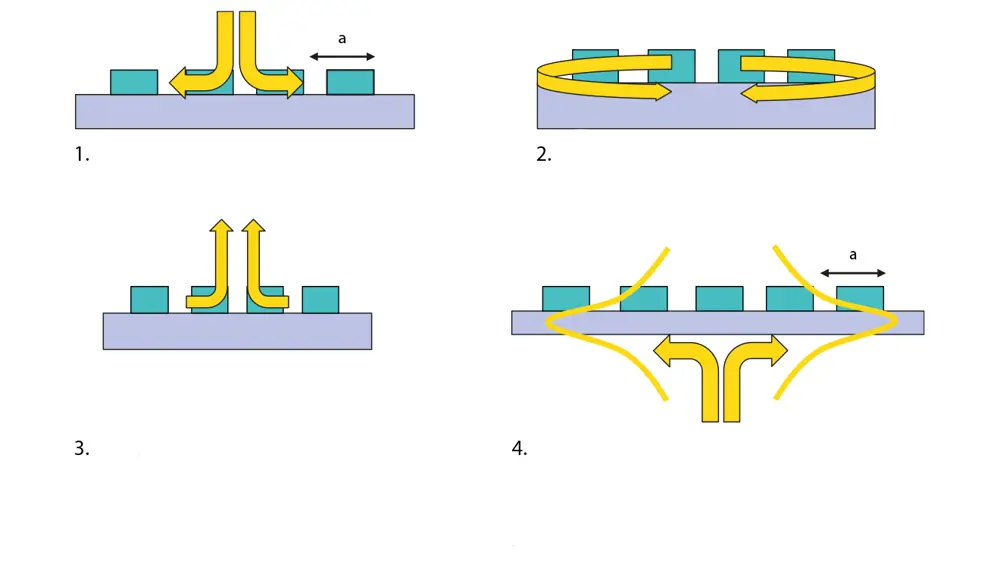
1) Light enters the diffraction grating layer of the sensor. 2) Light becomes trapped in a surface resonance. 3) Light is reflected to the sensor, in the form of a strong localised wave of energy that can be seen as a signature sharp peak in the reflected spectrum. 4) Some of the light interacts with the environment beyond the sensor. If the refractive index changes (for example, due to the presence of a biofilm), this causes a shift in the peak of the reflected spectrum.
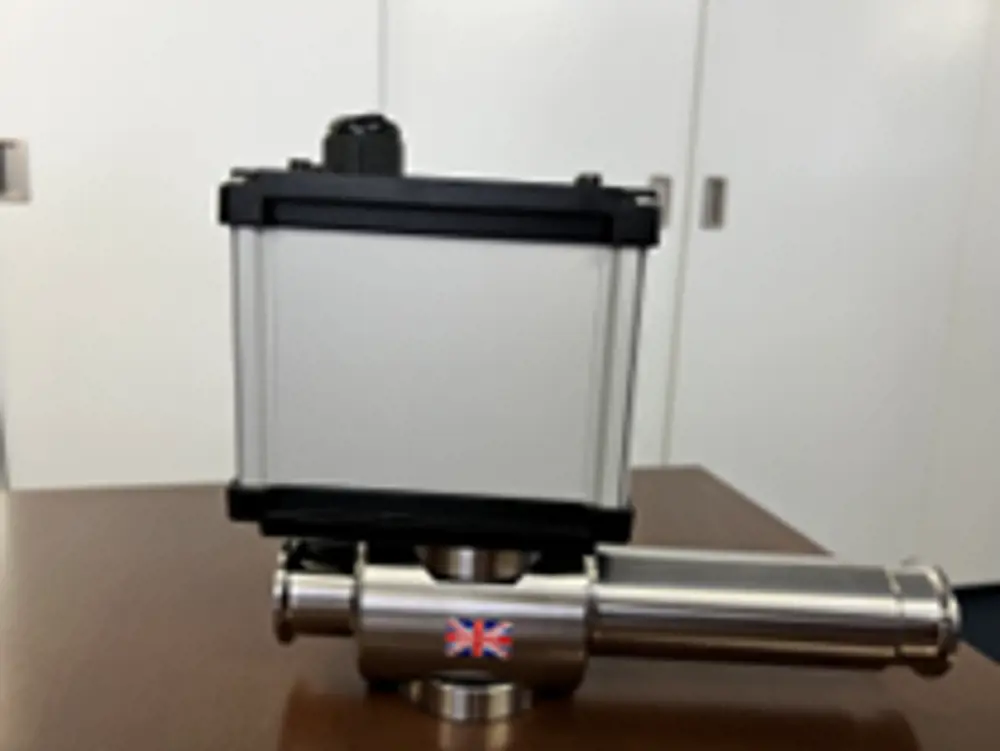
The sensor instrument mounted on a flow-through pipe. These pipes are used throughout the plant to transport liquids between tanks, from tanks to mixers, and eventually to the bottling unit © Phil Marsden
Real-world testing
Integrating a device developed in an academic setting into the industrial manufacturing process is challenging. The new technology needs to be as reliable or better than existing methods before manufacturers can complete the transition.
The first phase of the project installed the new sensor technology in a controlled environment at P&G’s research labs in Reading. P&G’s microbiologists intentionally added bacterial spikes with independent experts testing whether the sensors could detect biofilms with the required sensitivity and thus provide early warnings of microbial contamination. The sensors consistently performed well in laboratory tests and were verified by P&G at the pilot plant scale, so now the project has moved to the manufacturing demonstration phase.
According to Andy Dorset, Senior Director of Quality Innovation at P&G, “the manufacturing demonstration phase marks an exciting moment for using real-time in-line sensors to detect microbial contamination throughout the entire supply chain, from suppliers to export trucks. These sensors, connected to a digital platform, can provide insights into how the different parts of the process can potentially impact microbial contamination.”
P&G has installed multiple sensors to monitor the presence of microbes at different stages of the end-to-end Fairy liquid supply chain. The sensors have been implemented at an external supplier in Manchester, as well as throughout the product manufacturing process in London and within trucks that transport intermediate materials to a central European location, where the product is bottled.
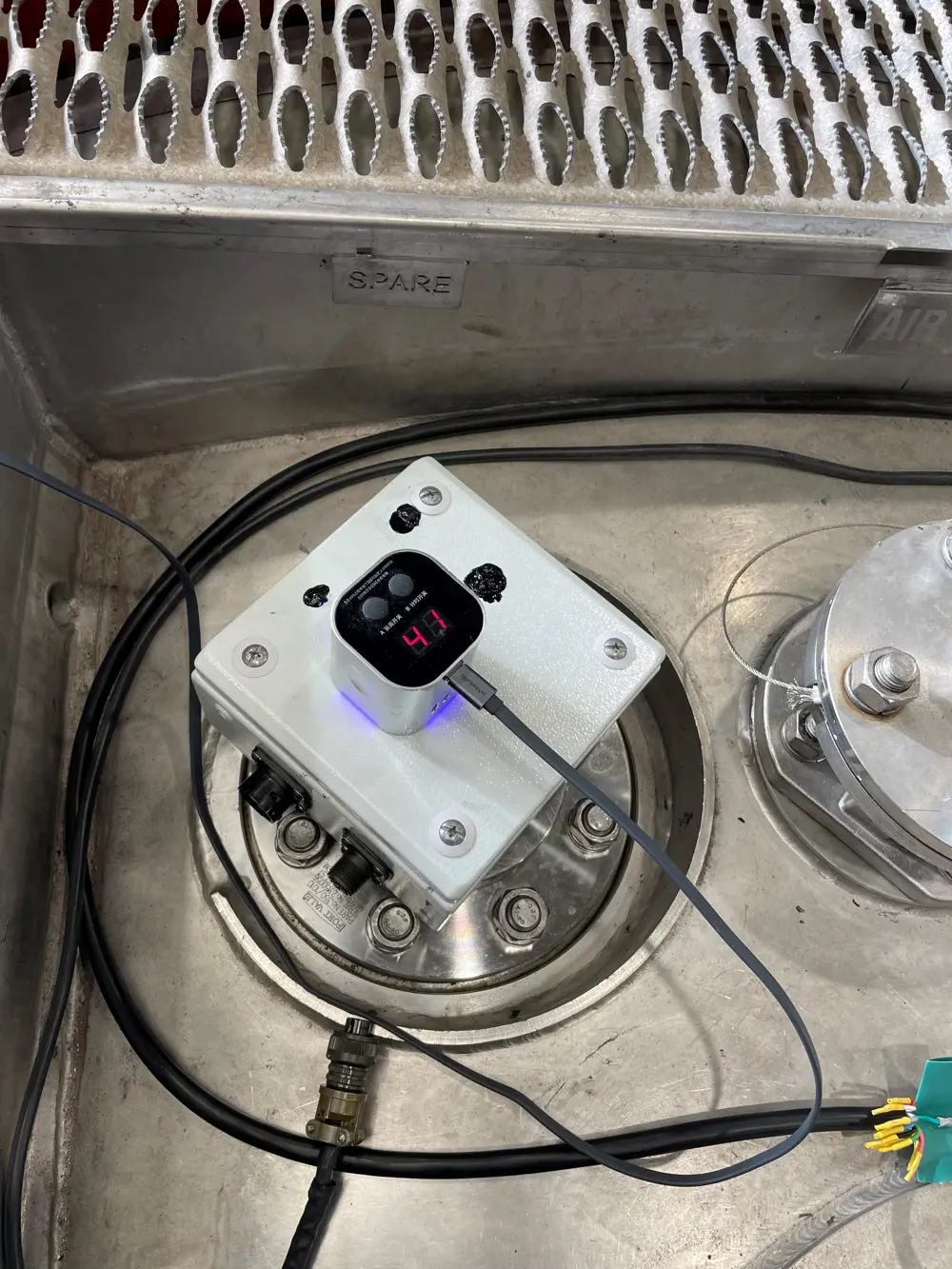
The sensor installed at the top of liquid storage tanks. Installing the sensors in hard-to-reach areas such as these is particularly beneficial. Condensation can form at the top of storage tanks, which could promote more bacterial growth that may otherwise not be detected © Phil Marsden
Dorset notes the potential of these sensors to replace the current manual methods, describing these as “labour-intensive and sample-heavy”. He emphasises that “if successful, this sensor-based approach would provide information much earlier than manual methods. It would enable faster decision-making to address any microbial issues, and also lead to productivity gains by digitising manual and paperwork-intensive processes.”
It will take time to complete this phase of the project and the sensors must be able to alert the company to unplanned contamination on the production line to prove successful. P&G has to establish the effectiveness of the sensor-based approach compared to the existing manual microbial analysis before it can consider a complete replacement of the current process.
Dorset further explains that the new approach could affect the organisation’s cleaning and sanitisation frequency. “The sensors would serve as in-line monitors, notifying us when we need to take action instead of following a fixed schedule.” He adds: “With these benefits in mind our intention is to expand the implementation of these sensors and digitisation to encompass our microbe-susceptible supply chains. This is a pioneering step forward in ensuring product quality in our supply chain.”
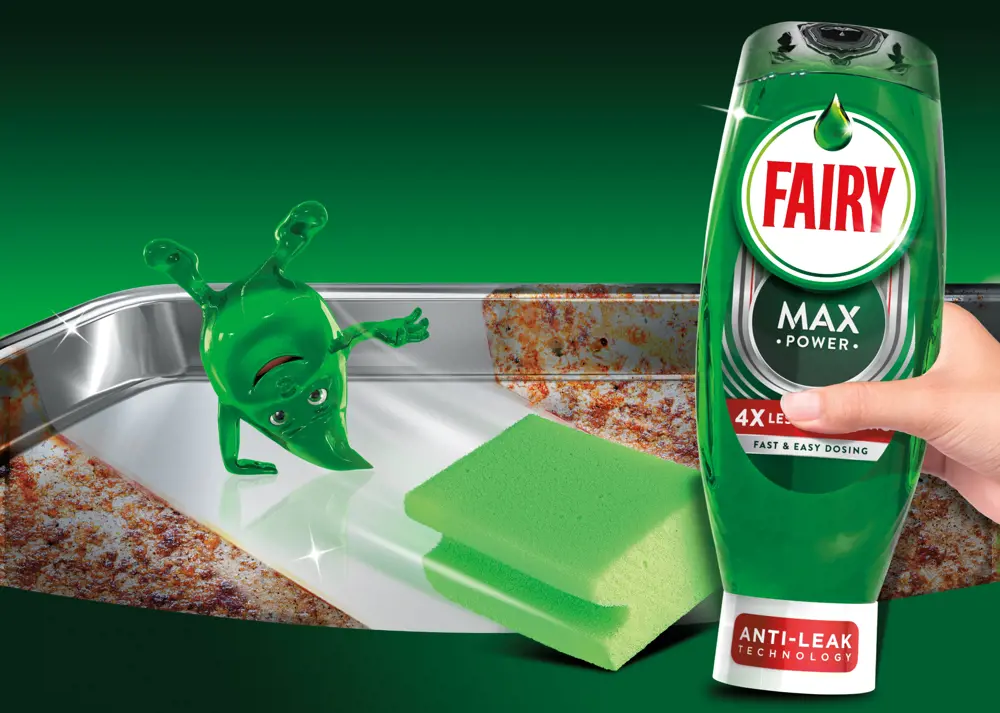
© P&G
Beyond washing up
The York research group has founded a spinout company, Phorest Diagnostics (drawing inspiration from the word ‘photonics’ – the study of light), to continue developing its patented chirped-GMR technology. This will also allow them to draw on the challenges and learning experience encountered so far.
“Installing the sensor in an industrial setting has been a steep learning curve. We needed to consider not only the robustness of the technology, but also its interfacing with the process control software” explains Professor Thomas Krauss. “Moreover, all the fittings needed to meet Clean Design specifications, which is not something we worry about in the academic setting.”
Phorest Diagnostics aims to expand into other industrial sectors beyond washing detergents where in-line bacterial monitoring is essential, such as food production and transport.
“Looking ahead, we are very excited about the prospect of a fully digital supply chain, where process engineers can remotely monitor the quality of product end-to-end, from precursor to finished product,” Krauss adds. “To this end, we have already installed a sensor on the truck of a major logistics company.”
As industries continue to embrace smart manufacturing, innovations such as the chirped-GMR sensor will play a crucial role in enhancing efficiency, reliability, and overall productivity.
Contributors
Andy Dorset is Senior Director, Quality Innovation, at P&G’s Newcastle Innovation Centre. He led the funding call with Innovate UK and created the ARGUS consortium. In combination with a P&G team of microbiologists, digital experts and process engineers, this consortium created the first manufacturing demonstration prototype – connecting microbial contamination sensors digitally across the end-to-end Fairy liquid supply chain.
Professor Steve Johnson is Professor in Engineering at the University of York. He leads research into technologies that integrate functional biological molecules with solid-state systems, such as electronic or photonic devices. He has a particular interest in the development of these hybrid technologies to address societal challenges in healthcare and sustainability.
Professor Thomas Krauss is Professor of Photonics at the University of York. He runs a 15-strong group working on photonic nanostructures, how they can be used to manipulate light and how they can interrogate biological processes. He is the inventor of the chirped guided-mode resonance technology used to detect biofilm growth.
Keep up-to-date with Ingenia for free
SubscribeRelated content
Design & manufacturing

Super cool(er)
Welsh startup Sure Chill has developed a cooler that uses the properties of water to keep its contents cool for around 10 days without electricity. This is ideal for storing items such as vaccines where electricity sources are unreliable.

R&D investment makes good business sense
In just five years, Dr Ralf Speth FREng has presided over a revolution in design and manufacturing that has helped create a new family of engines and has overhauled Jaguar Land Rover (JLR) production facilities.

Steel can arise from the ashes of coal
Thousands of people were laid off in the UK steel industry in 2015 and there are pessimistic future forecasts. Professor Sridhar Seetharaman of the Warwick Manufacturing Group argues that smaller, flexible steel mills implementing new technology would better cope with fluctuating global trends.

Integrating metrology in business and academe
Professor Jane Jiang’s interest in measuring began when she worked on a bus production line in China. She found that the best way to improve quality, consistency and productivity was through metrology, the science of measurement. Today, she runs the UK’s largest metrology research group.
Other content from Ingenia
Quick read
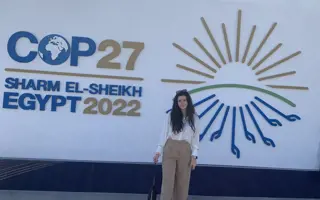
- Environment & sustainability
- Opinion
A young engineer’s perspective on the good, the bad and the ugly of COP27
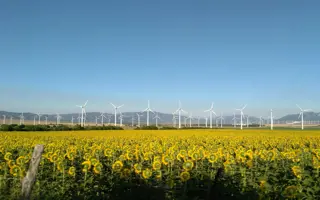
- Environment & sustainability
- Issue 95
How do we pay for net zero technologies?
Quick read
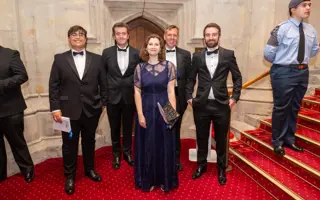
- Transport
- Mechanical
- How I got here
Electrifying trains and STEMAZING outreach
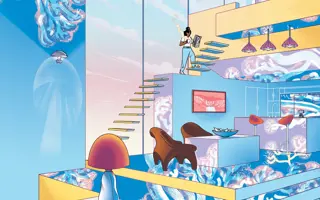
- Civil & structural
- Environment & sustainability
- Issue 95