Stabilising the London Millennium Bridge
When it opened to the public on 10 June 2000, the Millennium Bridge exhibited large sideways movements. Subsequent research and testing showed that the movement is unrelated to the particular form of the Millennium Bridge, and that many other bridges would move in the same way under similar crowd loading. The movement is caused by a little-known phenomenon called synchronous lateral excitation, due to overcrowding by pedestrians.
This article describes the design of the bridge, the events surrounding opening day and the results of the research and testing, and gives an explanation of the modification works currently being installed.
In September 1996 a competition was organised by the Financial Times in association with the London Borough of Southwark to design a new footbridge across the Thames. The competition attracted over 200 entries and was won by a team comprising Arup (engineers), Foster and Partners (architects) and the sculptor Sir Anthony Caro. The bridge was then commissioned by the London Borough of Southwark in association with the Millennium Bridge Trust and the Corporation of London.
Design and stiffness
The Millennium Bridge is conceived as a ‘minimal’ way of providing a connection between the two banks of the Thames. A group of cables either side of a deck span the river over two river piers to form a shallow suspension bridge. The rise from the lowest to the highest point of the cables is 2.3 m over the 144 m centre span. The slender structure that results from this concept enables the bridge to fit within the tight constraints imposed by the river navigation channel beneath the bridge, and the St Paul’s height restrictions above the bridge.
The bridge is 333 m long overall in three spans: north 81 m, centre 144 m, south 108 m. The cables are fixed at each bank on to a thick concrete slab anchored by large-diameter concrete piles. The river piers are supported by 6 m diameter concrete caissons excavated to 18 m below the river bed.
The dead-load of the bridge is 2 tonnes per metre along the bridge axis. The shallow rise of the suspension cables gives a high resulting dead-load cable tension of 22.5 MN; these large cable tensions are used to provide the stiffness to the structure. Accordingly, the stiffness of the structure is almost identical laterally (side to side) and vertically.
Plan bracing to form a lateral girder beneath the deck was considered during the design of the bridge, but adds little stiffness in comparison with that provided by the cables. The graph of lateral frequencies of a selection of footbridges shows that the lateral frequencies of the Millennium Footbridge are similar to those of other bridges.
Opening day
The bridge opened to the public on 10 June 2000. Up to 100,000 people crossed it that day with up to 2000 people on the bridge at any one time.
When large groups of people were crossing, greater than expected sideways movements occurred. These movements were not continuous; they occurred when a large number of pedestrians were on the bridge and died down if the number of people on the bridge reduced or they stopped walking. The movement was limited by the fact that beyond a certain level, a significant number of pedestrians began to have difficulty in walking and held on to the balustrades for support.
The same phenomenon was not observed with any vertical modes. The bridge behaved vertically as predicted in the design, that is, the vertical movements that took place were within the limits of normal good practice.
In order to fully investigate and resolve the lateral movements, the decision was taken to close the bridge on 12 June.
Synchronous lateral excitation
The Millennium Bridge movement was caused by sideways pedestrian loads exerted in the following way. A random event such as chance correlation of the pedestrians’ steps causes slight sideways movements of the bridge. It becomes more comfortable for the pedestrians to walk in synchronisation with the bridge movement (hence, ‘synchronous excitation’). This instinctive behaviour ensures that the lateral forces exerted by the pedestrians match the resonant frequency of the bridge, and are in phase such as to increase the motion of the bridge. As the amplitude of the motion increases, the total lateral force increases and becomes more correlated.
The research programme
In order to design a method of reducing the movements, the force exerted by the pedestrians had to be quantified and related to the motion of the bridge. Although there are some descriptions of this phenomenon in existing literature, none of these actually quantifies the force. So there was no quantitative analytical way to design the bridge against this effect. We therefore decided to undertake a programme of research during the summer of 2000 following the bridge closure.
We carried out laboratory tests involving pedestrians walking over moving surfaces at Imperial College, University of London, and at the Institute of Sound and Vibration Research at Southampton University. In addition to this we felt that the only way to replicate the precise conditions of the Millennium Bridge properly was to carry out crowd tests on the bridge deck itself. The first of these was carried out with 100 people in July 2000.
Test results
We calculated the sideways force exerted by the pedestrians on the structure using the results from the crowd tests on the bridge. The movement of the bridge under the pedestrian force was measured using accelerometers and these results were then processed to determine the forces involved. The test results show a clear relationship between the movement of the bridge and the force exerted by the pedestrians, which can be expressed as
F = K x V
where F is the average sideways force exerted on the bridge by a pedestrian walking on the deck, K is a mathematical constant and V is the sideways velocity of the bridge.
Using this formula, it is possible to calculate the number of pedestrians that will cause excessive sideways movement.
The effect can occur on any type of bridge structure with a lateral frequency of less than around 1.3 Hz, a limit arising from the maximum normal pacing frequency of pedestrians. The number of pedestrians required is then related solely to the mass of the bridge, its frequency and its damping.
Comparison of results with bridge behaviour
The test results bore out the observed behaviour of the bridge on opening day in two significant ways.
First, and perhaps most surprisingly, during the tests we found no lower limit to the frequencies that could be affected by synchronous lateral excitation. Rather we found that, the lower the frequency, the more damping would be required to counteract the phenomenon. This discovery is consistent with the bridge behaviour observed on opening day. The sideways movements occurred more often on the south span at a frequency of 0.77 Hz and in the first lateral mode of the central span at a frequency of 0.49 Hz; they occurred less often on the north span which has a first lateral frequency of 1.05 Hz.
Secondly, the results indicate that pedestrians work as ‘negative dampers’, acting to reduce the inherent structural damping of the bridge. This explains the observed ‘on–off’ nature of the movement. A critical number of people is required to reduce the inherent structural damping of the bridge to zero. Until this critical number is reached there is no evidence of the effect and the movements of the bridge are low. If this number is exceeded, the force input is greater than the bridge structural damping, and the movement will quickly begin to increase.
Since the force exerted by the pedestrians is proportional to the bridge movement, the force will increase as the movement increases, and this in turn will increase the movement. This positive feedback loop causes the lateral motion to increase until the force exerted by the pedestrians is limited by their ability to walk normally.
Other bridges
During our investigations we found that other bridges – with completely different structures to the Millennium Bridge – have also moved laterally under large crowds. An example of this is the Auckland Harbour Road Bridge which has a box-girder structure. This bridge was filmed vibrating laterally at 0.7 Hz when a large crowd of demonstrators walked over it in 1975.
This is particularly significant because the Auckland Harbour Bridge is a large road bridge with a conventional structure. These cases of lateral vibration have not been widely published and as a result the phenomenon has not become known to practising bridge engineers, nor adequately researched.
Modifications to the Millennium Bridge
Unless usage of the bridge was to be greatly restricted, we considered that there were only two feasible, generic options to improve its performance. The first was to increase the stiffness of the bridge to move its natural frequency out of the range that could be excited by the lateral footfall forces; the second was to increase the damping of the bridge such that the resonant response was reduced.
The stiffening option
Synchronous lateral pedestrian loading is now believed to be possible at any frequency below about 1.3 Hz. Therefore, to avoid the possibility of resonant structural response, all modes with a significant lateral component would need to be moved above this value. The most difficult mode to stiffen is the first lateral mode of the centre span of the bridge, which has a natural frequency of 0.49 Hz, thereby requiring approximately a threefold increase. To achieve a threefold increase in natural frequency the lateral stiffness must be increased by a factor of nine, on the assumption that this can be done without increasing the mass at the same time. In practice any stiffening scheme will also involve increasing the mass so the stiffening factor required is in fact well in excess of tenfold.
The geometric tension stiffness of the main cables provides a high inherent stiffness to the structural system. Providing additional stiffness by more conventional means, such as fully bracing between the edge tubes of the deck, would only increase overall stiffness by a few percent. Therefore to achieve an increase of around ten in stiffness would require considerable additional structures. Additionally, the lateral-torsional modes of the structure would require extra, separate structures to be added.
For these reasons a stiffening option, whilst feasible, would be intrusive, complex and time-consuming and so was not favoured once we had proved that a damping-based solution was feasible.
Design of damping solution
The amount of damping required on a bridge is proportional to the number of simultaneous users for which it is to be designed. Since the Millennium Bridge is expected to be a popular location for crossing the Thames, we decided on a design level of 2 persons per square metre over the whole bridge for the design of the damping. This compares to a figure of around 1.3 to 1.5 persons per square metre seen at the peak on opening day. The testing that we carried out also showed that, above a density of around 1.8 persons per square metre, the forces imparted are reduced due to the difficulty people experience in walking in such tightly packed crowds.
The relationship of the input force to the velocity of the bridge results in the greatest damping requirement at the lowest frequency. The first lateral mode of the central span has a frequency of 0.49 Hz which results in a damping requirement of over 20% of critical damping. Since such large damping levels are difficult and expensive to achieve, the choice of pedestrian design load is critical and will require significant discussion on future projects.
The lateral damping for the Millennium Bridge is provided primarily by fluid viscous dampers. A total of 37 viscous dampers are to be installed, the majority of which are to be situated beneath the bridge deck, on top of the transverse arms, every 16 m. Each end of the viscous damper is connected to the apex of a steel V-brace, known as a chevron. The apex of the chevron is supported on roller bearings that provide vertical support but allow sliding in all directions. The remote ends of the chevron are fixed to the neighbouring transverse arms. In this way the lateral modal movement over 16 m is mobilised to ensure maximum movement at each damper.
Where possible the viscous dampers are connected to fixed points such as the piers and the ground. This makes the dampers considerably more efficient since the movement is transferred directly into the damper stroke rather than via a structure which is itself subject to deformation. There are viscous dampers in the plane between the cables and the deck at the piers. These provide vertical and horizontal damping. In addition, a pair of dampers are located to each side of the approach ramp on the south abutment. These provide damping primarily for the lateral and lateral-torsional modes on the south span.
As stated earlier, we have no evidence of vertical synchronous excitation. However, other researchers believe they have and therefore as a measure of risk reduction we are also installing tuned mass dampers for the vertical modes around the range of walking frequencies. The tuned mass dampers are arranged in pairs on each transverse arm.
The modification works are now underway and the Millennium Bridge is scheduled to reopen at the end of 2001.
Conclusions
The phenomenon of synchronous lateral excitation seen on the opening day was not related to the technical innovations used in the design of the Millennium Bridge. The same movement could theoretically occur on any future or existing bridge with a lateral frequency under approximately 1.3 Hz, with enough pedestrians on the bridge in relation to its mass and damping.
The non-linear nature of the effect means that satisfactory past performance of a bridge under less than critical loading is not a guide to its future performance, should the number of users reach the critical value. We believe that knowledge of the loading condition should be disseminated widely and a check for this effect should be incorporated in standard bridge design procedures. This will require sensible standards to be set for numbers of users in order to avoid unnecessary extra costs. There is obviously a great deal of further research to be done to fully define and quantify this effect for general application.
Further reading
Linking London: The Millennium Bridge (2001). Tony Fitzpatrick, Pat Dallard, Sophie Le Bourva, Angus Low, Roger Ridsdill Smith, and Michael Willford. (Includes a section describing the analytical research carried out.) Available from The Royal Academy of Engineering (contact Eda Ramadan: ramadane@raeng.co.uk) or from the authors.
Tony Fitzpatrick FREng
And Roger Ridsdill Smith Ove Arup Partnership
Tony Fitzpatrick is a structural engineer who joined Ove Arup after graduating from university in 1972. He became a director of Ove Arup & Partners in 1986, joined the Main Board in 1994, and is currently Chairman of Arup’s Americas Division. He has worked on major projects around the world, with particular experience in tall structures and those with a major urban impact.
Roger Ridsdill Smith worked for four years in Paris before joining Ove Arup & Partners where he is an Associate Director. He was joint winner of the Institution of Structural Engineers 1994 Design Competition for Young Engineers, and nominated as one of Building magazine’s ‘Forty under Forty’ most promising construction professionals in July 2000. Roger has worked on a range of high-profile structures in the United Kingdom, France, Spain and Germany.
Email: Tony.Fitzpatrick@arup.com; Roger.Ridsdill-Smith@arup.com
Keep up-to-date with Ingenia for free
SubscribeOther content from Ingenia
Quick read
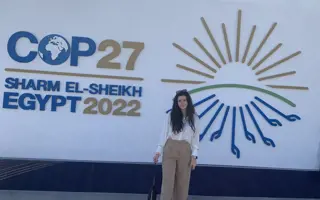
- Environment & sustainability
- Opinion
A young engineer’s perspective on the good, the bad and the ugly of COP27
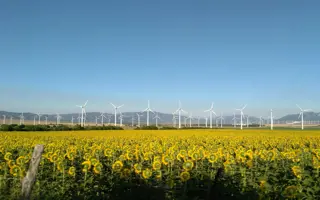
- Environment & sustainability
- Issue 95
How do we pay for net zero technologies?
Quick read
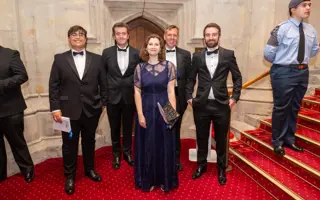
- Transport
- Mechanical
- How I got here
Electrifying trains and STEMAZING outreach
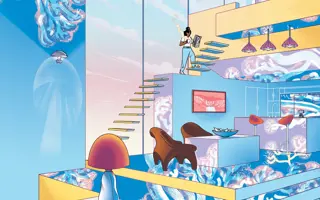
- Civil & structural
- Environment & sustainability
- Issue 95