Satellite broadband around the globe
Satellite broadband around the globe

2010 MacRobert Award winner, Inmarsat, for developing a lightweight and portable mobile satellite communication system
Inmarsat was awarded The Royal Academy of Engineering MacRobert Award in June 2010. The company won the £50,000 prize for the development of its broadband global area network system, known as BGAN. Dr Rebecca Pool talked to members of the Inmarsat development team about how they created a lightweight and portable mobile satellite communication system that can fit into a backpack.
Founded in 1979 as an intergovernmental organisation (IGO), the International Maritime Satellite Organisation was established to set up a free satellite communications network for the maritime community. Providing critical communications to around 900ships in the early 1980s, the company grew to offer similar services to other users on land and air and in 1999 became the first IGO to be transformed into a private company, Inmarsat.
Now a publicly-traded company, Inmarsat still provides its Global Maritime Distress and Safety Service as a public service to the maritime community as well as satellite-aided air traffic control to the aviation industry. However, the company also supports voice and high-speed data links to more than 287,000 ship, vehicle, aircraft and other mobile users in remote regions. These services are delivered through 11 geostationary satellites, controlled from the company’s London headquarters.
One key service is the broadband global area network (BGAN), which represents an ingenious example of systems engineering. Come the mid-1990s, the company wanted to provide global mobile broadband services, that is, deliver digital voice and data services, at around 384 kbps: to anywhere in the world, consequently BGAN was conceived.
The satellite communications network currently comprises three geostationary satellites, several land-based earth stations and a terrestrial communications network. It can be accessed via battery-powered, briefcase-sized terminals anywhere in the world except at the poles. If users want to receive or send voice or data messages, they simply connect a mobile phone or laptop to the satellite terminal, via an ethernet cable, USB cable or wireless bluetooth. As long as the terminal is in line-of-sight with one of the three satellites, the broadband communications network will be accessible.
While using BGAN is straightforward now, the technological barriers to launching the global mobile broadband service seemed insurmountable at first.
Fifteen years ago, satellite terminals were the size of small cars while Inmarsat services occupied little more than one third of the total 33 MHz of radio spectrum designated for all satellite operators. This limited bandwidth had primarily been intended for a small amount of voice traffic, a far cry from the ambitious 384 kbps data rates required for broadband communications. If BGAN was to succeed, a large range of issues had to be addressed.
Third generation solution
When designing the broadband network, the systems engineers first decided to adapt technology from so-called third generation or ‘3G’ mobile telecommunications. At the time this was neither a finished nor approved standard, but as it was already developed, it would save the business £1 billion in non-recurring engineering costs. However, to accommodate differences between a terrestrial and satellite mobile broadband network, and ensure that voice, data and multimedia services could be supported in a similar way to a terrestrial 3G network, the systems engineers made several changes.
A key challenge was to optimise the spectrum efficiency of the communications network, that is, maximise the information rate that could be transmitted over the given bandwidth. This would enable a greater quantity of voice and data traffic to be simultaneously supported by the network. The company’s team first bought an off-the-shelf core network from Ericsson, which formed the backbone of the communications network, primarily switching and routing calls across the system. The team went on to substitute the core network’s standard 19.2 kbit/s voice encoder, or vocoder, with a lower bit rate, 4 kbit/s vocoder. Compressing the voice channel in this way left more bandwidth to deliver other services, such as data transmissions.
With the core network in place, the engineers then designed a bespoke 3G radio access network, called BGAN Inmarsat Air Interface-2, to allow users to connect to the core network. Taking advantage of recent digital signal processing technologies, the engineers deployed, for the first time in a satellite network, the most efficient spectrum re-use technique available to convey data, 16 Quadrature Amplitude Modulation (QAM).
Now commonly used in digital terrestrial television such as Freeview, this digital modulation scheme is a form of so-called phase shift keying, developed to optimise data communications. Here the phase of the carrier signal is altered so data can be carried in a radio communications signal more efficiently, allowing higher data rates to be carried within a given bandwidth.
As well as the latest modulation scheme, engineers also adopted the most advanced method of forward error-correcting coding, Turbocode. This coding ensured the modulated transmissions could be reliably transferred across the communications system, especially in the presence of data-corrupting noise in space, at rates of up to 432 kbit/s. In addition to adopting these digital signal processing techniques, the Inmarsat team incorporated more sensitive receivers to the radio access network, to accommodate the lower receive signal power.
Satellite communication is renowned for its latency issues, typically a 250 ms delay between requesting data and the receipt of a response. However, the terrestrial 3G network had already been designed to accommodate latency delays of between 700 ms and 100 ms, so addressing satellite latency was relatively simple. Low latency communications links were substituted for high latency links in the digital communications network to enable speedier connections.
Satellite power
While the core network and radio access network had been re-developed to support more voice and data traffic within the limited bandwidth, more changes would be required if this part of the communications system was to operate in the satellite broadband network. Both the sensitivity of the receiving ground equipment and the radiated power of the satellite would have to increase, while the satellite would have to transmit data more efficiently.
Ground-based 3G networks comprise hundreds of thousands of base stations, receiving and transmitting radio frequency signals to mobile phone users or fixed phone networks over relatively short distances. In contrast, the satellite network would have to operate with just three satellites, serving as base stations, receiving and transmitting radio frequency signals through the electronically disruptive environment of space to satellite terminal users some 22,000 miles away on Earth.
Increasing the sensitivity of ground equipment was relatively straightforward: a larger antenna was added – some 15 to 19 m in length – to receiving satellite land earth stations. However, increasing the radiated power of the satellite as well as its data transmission efficiency demanded several breakthroughs. The end result was the new generation of satellite, the Inmarsat-4 (or I-4).
A first, relatively easy step in increasing the satellite’s radiated power was to incorporate more sophisticated solid-state power amplifiers. However, to boost power further and optimise data transmission, the satellite required a much larger antenna reflector than the previous generation’s 3 m diameter solid dish.
To this end, the Inmarsat team adopted a ‘mesh deployable’ design, originally developed for US intelligence in military missions. The antenna consisted of a network of folding graphite tubes that supported a lightweight mesh of gold-plated molybdenum wires. The wires were connected to a set of ties that held the mesh in the desired shape.
Before launch, the antenna was stowed in a cylinder 1 m in diameter and approximately 2 m long, within the satellite faring. Once the satellite was in orbit, the antenna was deployed by retracting the connecting cable, and the structure literally unfurled to yield a 9 m diameter reflector.
The larger diameter reflector was crucial to the satellite design as it allowed the system engineers to incorporate more spot beams to the satellite, increasing the effective radiated power of a satellite for a given amount of transmitting power. Satellites can transmit data signals as high power spot beams so that only terrestrial stations in the intended reception region will receive the full satellite signal.
Each of the company’s 11 satellites has global beam coverage in which a single global spot beam covers up to one-third of the Earth’s surface, apart from the poles. However, more recent satellites have multiple spot beams, each covering a fraction of the region covered by the global beam, but collectively providing global coverage.
Inmarsat’s third generation of satellite, the I-3, has a 3 m diameter reflector and seven ‘wide’ spot beams, while the I-4 satellites can transmit 19 wide spot beams and a further 228 narrow spot beams. This has contributed to the overall radiated power of an I-4 satellite being 60 times greater than an I-3, coming in at 67 dBW compared to 49 dBW for I-3.
More beams also means more efficient use of the radio spectrum, as each beam can be aimed at a different region, allowing the same radio frequency to be re-used to transmit different streams of data to several areas. So in the case of the I-4 satellites, each of the 19 wide beams and 228 narrow spot beams can be reconfigured and focused anywhere on Earth to provide extra capacity where needed. Indeed, compared to the I-3 satellites, this generation is 12 times more efficient in their use of the radio spectrum.
Satellite user terminals
A key achievement, instrumental to the satellite communications system, was to develop a portable satellite terminal. To do this, the engineers integrated a two to four patch L-band antenna to the terminal. This provided sufficient gain, at the given terminal size, to make efficient use of the relatively weak incoming radio frequency signals. To boost performance further, the terminal was also fitted with a more sensitive noise amplifier while a larger solid state power amplifier was used to increase transmit power.
But why not aim for a satellite-scale phone? Engineers realised from the outset that to deliver 432 kbps data rates to a device that could only house an antenna with 2 db or 3 db of gain, from a satellite many thousands of kilometres away, just wasn’t viable, especially when the laptop-sized terminal could deliver around 10 db more gain.
Summing up
The end result of the numerous technology breakthroughs and focused use of systems engineering is that, together, Inmarsat’s fourth generation satellites can support 16,000 voice calls simultaneously and support a maximum capacity of around 300,000 user terminals.
Twenty different satellite user terminals now exist that can operate in different environments and support broadband speeds up to 492 kbps. These can be used as part of the BGAN network to set up a temporary communications hub, providing a range of services including voice, email, web browsing and remote access to a company network.
In the recent Haiti disaster, the earthquake took out the existing terrestrial and cellular networks. Just days after the earthquake however, a total of 472 individual user terminals accessed the BGAN spot beam over the region. In one moment, 137 terminals, supporting many more individual users, were sending and receiving data while 35 telephone conversations were underway. During the course of 20 January 2010, it was calculated that the beam was used for a total 36,054 minutes of communication, with gigabytes of information transferred.
Inmarsat has now contracted US aerospace manufacturer Boeing to build the next generation of satellite, the Inmarsat-5. Three satellites will form part of a new US$1.2 billion worldwide wireless broadband network called ‘Global Express’.
Transmitting in the extremely high frequency Ka-band, each satellite will carry 89 Ka-band beams, allowing the network to offer download rates of 50 Mbps and upload speeds of 5 Mbps from mobile user terminals as small as 60 cm. The first of these satellites is scheduled for completion in 2013, with full global coverage expected by the end of 2014.
For further information see www.inmarsat.com
Keep up-to-date with Ingenia for free
SubscribeOther content from Ingenia
Quick read
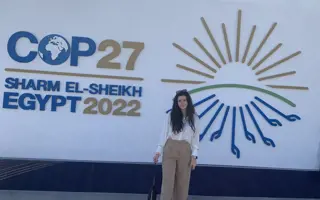
- Environment & sustainability
- Opinion
A young engineer’s perspective on the good, the bad and the ugly of COP27
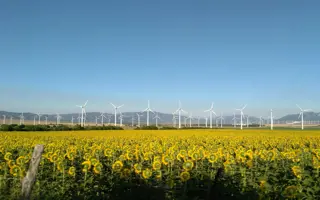
- Environment & sustainability
- Issue 95
How do we pay for net zero technologies?
Quick read
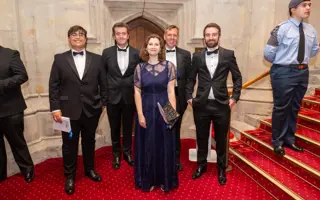
- Transport
- Mechanical
- How I got here
Electrifying trains and STEMAZING outreach
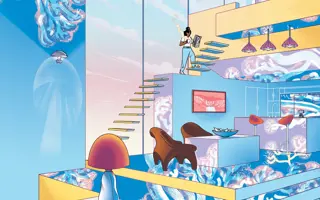
- Civil & structural
- Environment & sustainability
- Issue 95