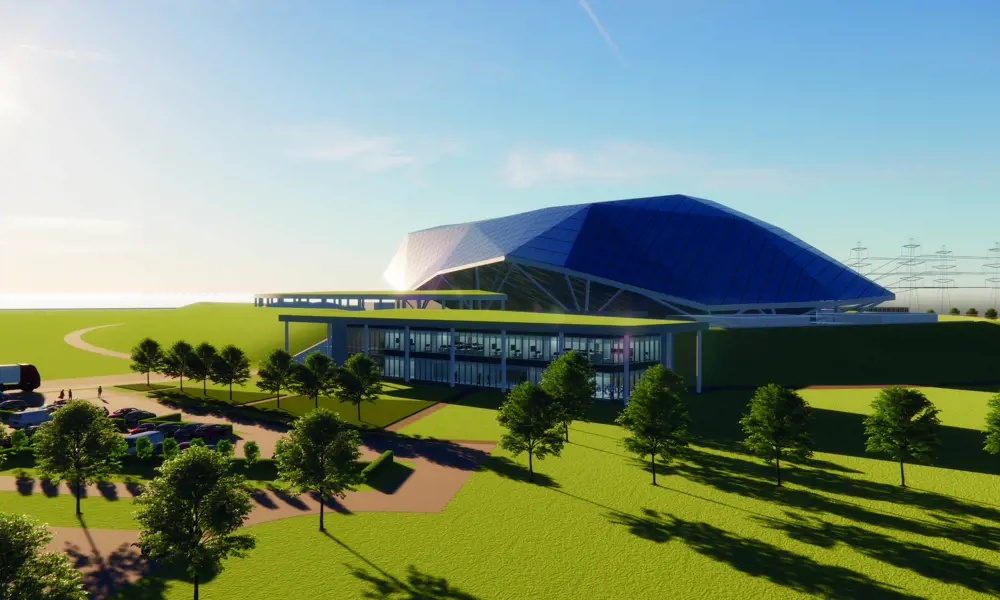
Nuclear designs on a low-carbon future
Did you know?
☢️ Fabrication and electricity production of small modular reactors (SMRs)
- SMRs produce up to 500MW of electricity
- An SMR has a footprint of about one and a half football pitches
- Around 90% of an SMR will be factory fabricated and moved to sites for assembly
- Modular design and a construction canopy reduce the risk of delays
The future energy market will be fundamentally different as the world eliminates carbon emissions in a move to an age of ‘net zero’. Cost-competitive, scalable and reliable sources of clean electricity will be a key element, both for direct use, as today, to charge electric vehicles, and potentially to power the production of synthetic fuels generated from hydrogen and captured carbon, to create fossil-free hydrocarbons as substitutes for oil and gas.
While new technologies can and must continue to be developed, we need to deploy existing low-carbon technologies more reliably and affordably to achieve net zero by 2050. We cannot rely on existing renewable technologies to fulfil those energy requirements alone because of their intermittency and the scale of the energy storage needed to accommodate their variability. For these reasons, wind and solar have significant limits. Nuclear power is increasingly seen as an integral solution for many countries and industrial users.
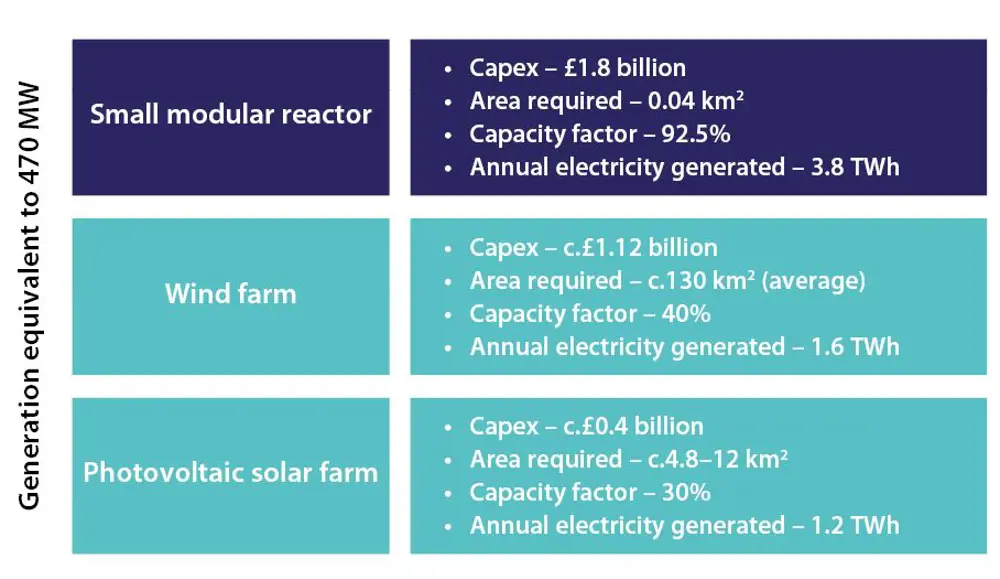
A small nuclear reactor would fit on an area smaller than two football pitches, less than land required for other fossil-free electricity generators
How SMRs can provide small-scale nuclear energy
Nuclear power currently produces around 10% of the world’s electricity. This power source can deliver safe, reliable low-carbon power consistently from relatively compact power stations to feed the electricity grid. Nuclear power stations can also provide heat and electricity to installations that can deliver other forms of decarbonised energy along with district heating. However, nuclear power has yet to be widely adopted. This is in part because of the high cost of the large individual power stations now on offer, and because the price of nuclear electricity from those facilities cannot compete with power from other sources.
Small modular reactors (SMRs) are a potential solution to this problem. The term SMR covers a range of differing nuclear technologies but it essentially refers to a newer generation of reactors designed to generate typically less than 500 MWe megawatts of electrical power, The idea is that factories will manufacture SMR components and systems for transport as modules for installation and commissioning at site. It is essentially a factory fabricated, road transportable and site assembled, fully integrated nuclear power station. The International Atomic Energy Agency (IAEA) currently lists over 70 different SMR designs under development using various technologies, including conventional water-cooled reactors, high temperature gas-cooled reactors, molten salt reactors, and micro-reactors, smaller devices with outputs of up to 10 MWe. There are also different approaches to manufacturing, installation and operation of these designs.
The key principle behind the design is to enable the safe delivery of low-carbon power at the lowest Levelised Cost of Electricity (LCoE) to the consumer
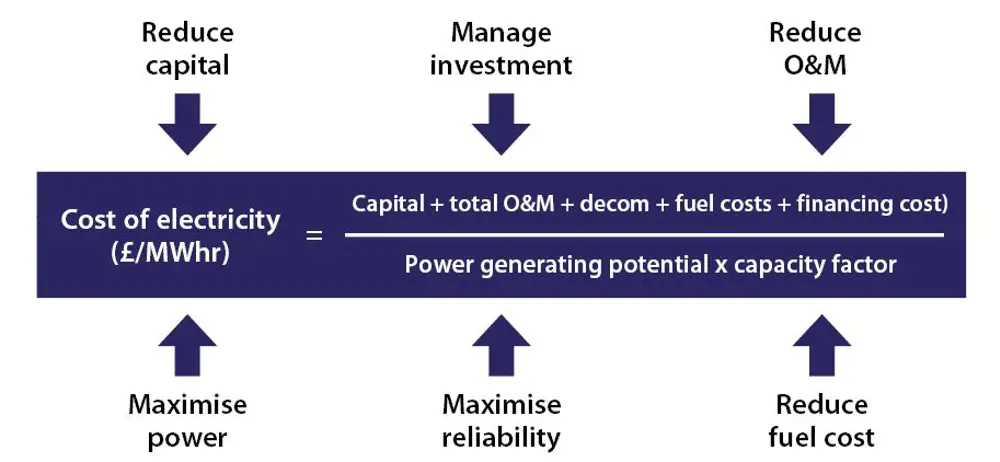
The Rolls-Royce-led consortium uses this equation to ensure that decisions across the SMR programme result in the lowest cost of electricity
A consortium led by Rolls-Royce has devised an SMR where each plant is around the size of one and a half football pitches. Its design is projected to cost £1.8 billion for a power station with an output of 470 MWe with a four-year build time. The key principle behind the design is to enable the safe delivery of low-carbon power at the lowest Levelised Cost of Electricity (LCoE) to the consumer. The LCoE is a commonly used way of comparing the costs of different ways of generating electricity. The LCoE incorporates energy projects’ complete costs and, for this UK-SMR programme, the LCoE also includes the cost of waste management and decommissioning. Each SMR aims to operate for 60 years at a LCoE (real delivered cost) between £35 and £50/MWh, roughly the current wholesale market price.
Innovative plant design to drive down Levelised Cost of Electricity (LCoE)
The consortium’s focus has been on aspects of plant design that impact LCoE. These include construction, capital costs, financing risk, build time, and operation and maintenance costs. This analysis has resulted in various decisions. These include the design not just of the nuclear island but of the complete power station. It also influenced the decision to base the design on a pressurised water reactor (PWR) and accompanying off-the-shelf fuel technology, using standard nuclear energy technology used in 400 reactors around the world. In this way it could ensure compatibility with existing regulatory processes and existing nuclear infrastructure around fuel and waste routes. The focus on LCoE also resulted in a decision to adopt factory fabrication and modularisation followed by plant assembly on an aseismic bearing – a giant shock absorber that neutralises local seismic conditions.
PWRs have been the predominant nuclear technology since the development of the first commercial plant in the 1960s. Various designs of PWR constitute the majority of the world’s nuclear power fleet, including the UK’s latest generation of reactors. The consortium saw this proven technology as the best option in combination with advanced digital, manufacturing and construction techniques. Operators and nuclear regulators globally understand the fundamental design of PWRs, reducing regulatory risk and the need for a prototype, further reducing cost and time to deployment.
UKRI anticipated that the LCN programme could yield a return £52 billion to the UK economy by 2050 if a full fleet of 16 power stations is built
Nuclear island
🌡️ How the pressurised water reactor-based technology forms the basis for the UK-SMR
The UK-SMR is built around a ‘nuclear island’ based on proven pressurised water reactor (PWR) technology, using industry standard uranium oxide (UO2) as fuel. Three centrifugal pumps circulate coolant to three corresponding vertical steam generators, with a total electrical output of around 470MWe. The design of the nuclear island also includes multiple active and passive safety systems, each with substantial internal redundancy.
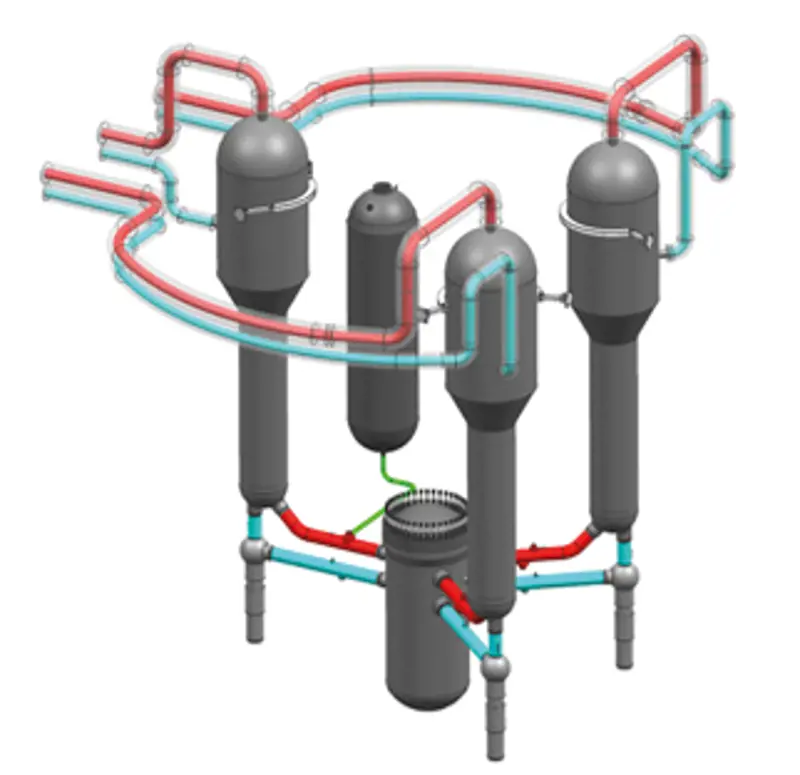
The nuclear island, a critical component of the power station, represents only about 25% of the overall cost. Therefore, to drive down the LCoE it is important to consider the design of the whole plant at the outset. For this reason, the consortium includes organisations from both the nuclear and civil construction sectors including Bam Nuttall, Laing O’Rourke, Atkins, Jacobs, Assystem, TWI, National Nuclear Lab (NNL), and the Nuclear Advanced Manufacturing Research Centre. The current phase of the programme has been jointly funded by all consortium members and UK Research and Innovation (UKRI). UKRI launched the programme in November 2019 with an initial investment of £18 million matched by the Rolls-Royce UK-SMR consortium.
UKRI increased its involvement in SMRs a year later with £215 million investment in the Low Cost Nuclear (LCN) programme, matched by £300 million from private funding sources. The aim of the LCN is to enable the UK to deploy SMR and advanced modular reactors quickly and efficiently. UKRI anticipated that the LCN programme could yield a return £52 billion to the UK economy by 2050 if a full fleet of 16 power stations is built. There could also be a £250 billion export market, creating up to 40,000 high-value jobs and rejuvenating UK manufacturing in the north of England and North Wales.
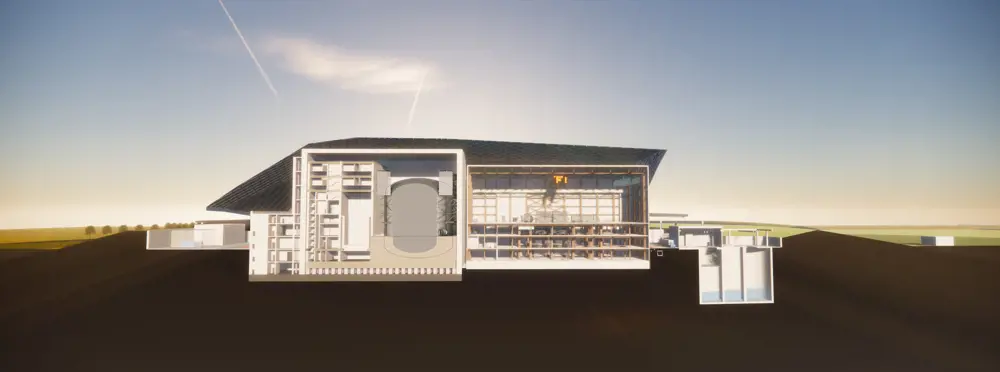
Optimising the use of floor space creates a compact building footprint. The UK-SMR fits into a single building with on-site storage to hold spent fuel for up to 100 years before it must be transferred to a long-term storage facility © UKSMR Consortium
The overall plant design includes several areas of innovation. For example, modularisation of the entire power station enables around 90% of the plant to be factory fabricated and road transported to site for assembly. The whole production process brings together advanced manufacturing techniques, with digital process control. The plan is to integrate this approach, often known as Industry 4.0, with advanced manufacturing methods such as additive layer manufacturing, or 3D printing, of metals, along with ‘inspect while weld’ techniques and large-scale robotic handling. In this way, we can bring to the nuclear industry techniques that have revolutionised the cost and ‘right first time’ rate of high-value manufacturing. These innovations, along with process monitoring including the use of Internet of Things and other techniques, will allow the efficient standardisation of the product, module sizes and interfaces. They will also enable factory testing and commissioning of individual modules prior to site assembly. Additionally, wherever possible modules are designed to incorporate ‘off-the-shelf’ products and to reduce the need to invest in specialist factory facilities.
The modular design aims to reduce the risk of construction delays, which can significantly increase costs and timescales at many nuclear new build sites. The compact layout for the UK-SMR design means that a construction canopy can be used for the first time to cover the entire nuclear construction site. This approach reduces the risks that adverse weather will delay construction. The canopy also provides internal craneage and an adequate construction environment day and night, if required. Many civil construction projects have successfully used this approach.
The compact layout is also designed to protect the reactor against seismic events. Aseismic bearings can isolate the nuclear power station from the ground and any seismic motion. An advantage of this approach is that the bearings can be ‘tuned’ to a site and its level of seismicity – the size, type and layout – removing the risk of the plant itself needing redesign to meet local seismic conditions.
modularisation of the entire power station enables around 90% of the plant to be factory fabricated and road transported to site for assembly
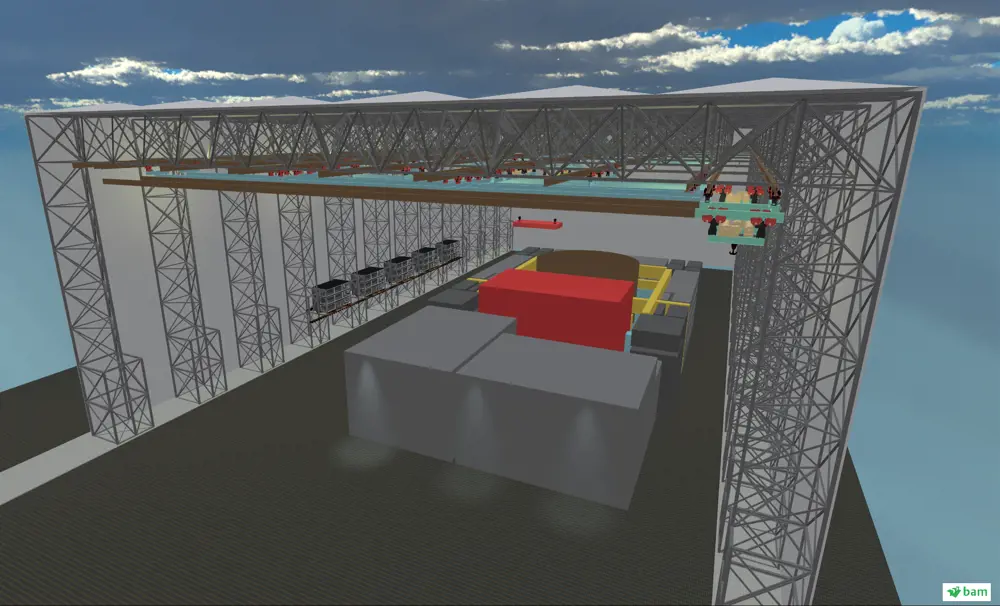
During construction, a canopy will cover the whole site, including cranes, to allow 24-hour operation and to reduce the risk that bad weather will cause delays © UKSMR Consortium
Digital twinning, building a virtual replica of the project as a way of monitoring progress and simulating the plant, underpins the design, construction and operation of the plant across all aspects of the programme. The system design will be maintained wholly digitally and integrated with analytical models for each element so at all times there will be a virtual plant on which design changes can be tested. As plants enter service, real-time operational data will be added continuously to provide the basis for intelligent maintenance and design improvements. Rolls-Royce has successfully applied these digital technologies on projects like large gas turbines, where engineers take entire digital twins from concept, through performance modelling, design, manufacturing, and on to in-service monitoring. The UK-SMR design has been digitally captured and will continue to be recorded through manufacture and construction to enable a full digital twin of each plant. This will not only allow simulation and optimisation of all stages of the plant lifecycle but is an essential component, enabling the plant to be successfully replicated at scale in other markets.
The fuel for the UK-SMR will use low-enriched UO2 assemblies with only minor modifications on those used to fuel conventional PWRs. Packaged in similar arrays, the fuel components for the UK-SMR will minimise development risks and costs. This choice means that existing commercial fuel manufacturers can supply fuel and that existing infrastructure for handling used fuel can be simply reused.
The emissions and waste from the SMR’s nuclear fuel cycle are clearly important. Over one year, a typical 500MWe coal-fired power station will burn around 1.6 million tonnes of coal and emit about 2.5 million tonnes of CO2. Over the same year, a UK-SMR could consume less than 16 tonnes of fuel and produce around 5m3 of higher activity radioactive waste, an amount that could fit into the back of a small van. Nuclear energy is the only power source that puts its waste in a container instead of into the atmosphere. Additionally, in line with current UK regulatory requirements, the UK-SMR is designed to store used fuel on site in purpose-built facility for up to 100 years before having to remove it to a long-term storage facility.
Producing affordable green power through UK-SMRs
The UK-SMR aims to reliably produce consistent low-carbon power at an affordable price. That it can be deployed at a wide range of appropriately licensed compact sites also means that it could be incorporated into an integrated, decarbonised energy system. Along with their key role as generators for the national power network, SMRs could also provide electricity for hydrogen, synthetic aviation fuel and other e-fuels. They could also have a role providing district heating to nearby towns and cities. This integrated approach means that a plant can be used at maximum efficiency alongside intermittent low-carbon technologies such as wind and solar. Alternatively, SMRs could be deployed remotely, connected to the grid through low-loss transmission technology or simply powering hydrogen or synthetic aviation fuel (SAF) plants where the fuel product can be transported to the point of use.
The UK-SMR aims to reliably produce consistent low-carbon power at an affordable price. That it can be deployed at a wide range of appropriately licensed compact sites also means that it could be incorporated into an integrated, decarbonised energy system
The process of production and assembly of the UK-SMR is designed to be scalable. To accelerate deployment, the factory and power station would be templates for reliable scale up without design changes or affecting quality. Essentially, we could build more factories where required if more power stations are needed. In this way, the UK-SMR could play in important role in the creation of integrated ‘energy parks’, supporting renewable sources of electricity in the creation of e-fuels for energy storage and transmission. Company nuclear power stations could also open up export opportunities. In the latest of several similar agreements, Rolls-Royce recently signed a Memorandum of Understanding with Fermi Energia to study the potential for the deployment of affordable, compact nuclear power stations in Estonia.
As the work continues, and to keep up the pace of progress, the UK-SMR team is making the transition from being a collaborative consortium to a standalone business, in which Rolls-Royce retains a significant interest, along with other equity investors. Work on the design, with more than 200 major engineering decisions made during the latest phase, has optimised the configuration, efficiency and performance criteria of the entire power station. In the process, it has increased the expected power capacity, without additional cost, from 440MWe to 470MWe. The plan is to have the first design to be assessed by regulators and to start the rigorous Generic Design Assessment process, through which the Office for Nuclear Regulation assesses whether the design meets all requirements for a nuclear plant to be operated in the UK, in the second half of 2021. This would keep the consortium on track to complete the first unit in the early 2030s and to build up to 10 by 2035.
***
This article has been adapted from "Nuclear designs on a low-carbon future", which originally appeared in the print edition of Ingenia 87 (June 2021).
Contributors
Sophie Macfarlane-Smith is responsible for the development of global customer opportunities and associated government relationships at Rolls-Royce. She completed a master’s in the physics and technology of nuclear reactors at the University of Birmingham and then joined Rolls-Royce’s reactor physics team in 1996. She has also had technical and project delivery roles in sectors including submarines, and naval and commercial marine. She has recently achieved her brown belt in Shotokan karate.
Paul Stein FREng is accountable for Rolls-Royce’s technology investment and ensuring close alignment with business strategy. He joined Rolls-Royce in 2010 as Chief Scientific Officer and acted as the Engineering and Technology Director for the company’s nuclear business for two years. Before joining Rolls-Royce, Paul was Director General, Science and Technology, at the Ministry of Defence. He is a Fellow of the Royal Aeronautical Society and the Institution of Engineering and Technology.
Keep up-to-date with Ingenia for free
SubscribeRelated content
Environment & sustainability

Recycling household waste
The percentage of waste recycled in the UK has risen rapidly over the past 20 years, thanks to breakthroughs in the way waste is processed. Find out about what happens to household waste and recent technological developments in the UK.

Upgrade existing buildings to reduce emissions
Much of the UK’s existing buildings predate modern energy standards. Patrick Bellew of Atelier Ten, a company that pioneered environmental innovations, suggests that a National Infrastructure Project is needed to tackle waste and inefficiency.

An appetite for oil
The Gobbler boat’s compact and lightweight dimensions coupled with complex oil-skimming technology provide a safer and more effective way of containing and cleaning up oil spills, both in harbour and at sea.

Future-proofing the next generation of wind turbine blades
Before deploying new equipment in an offshore environment, testing is vital and can reduce the time and cost of manufacturing longer blades. Replicating the harsh conditions within the confines of a test hall requires access to specialist, purpose-built facilities.
Other content from Ingenia
Quick read
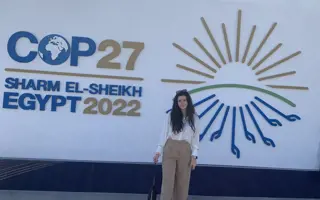
- Environment & sustainability
- Opinion
A young engineer’s perspective on the good, the bad and the ugly of COP27
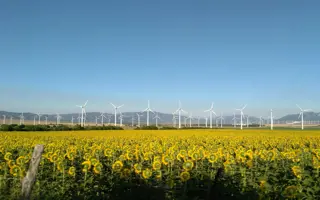
- Environment & sustainability
- Issue 95
How do we pay for net zero technologies?
Quick read
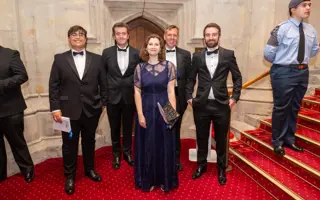
- Transport
- Mechanical
- How I got here
Electrifying trains and STEMAZING outreach
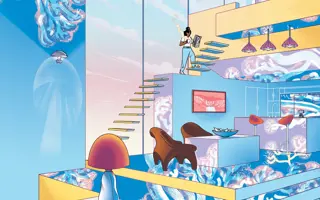
- Civil & structural
- Environment & sustainability
- Issue 95