
Mining volcanoes for metals
Did you know?
✨ Briny things
- Mining is the extraction of valuable geological materials and minerals from the Earth’s surface
- Volcanoes include a layer of brine, which could provide metals needed for our electronic devices and the energy transition
- Engineering has a key role to play in mining these brines
Generating and storing electricity requires materials with particular magnetic properties, such as rare earth metals for the static magnets in wind turbines. Perhaps most urgently, we need lithium and cobalt, which are essential components in batteries. While we have previously obtained these materials by mining, we are now acutely aware of how this industry can harm the environment: we need new sources and ways to find them.
The seabed is one potential source of minerals – geologists have found nodules composed of oxides of manganese and iron, often mixed with compounds of other elements. However, obtaining these would involve dredging the seabed, which poses a threat to local ecosystems, and the whole idea of ‘seabed mining’ has become a controversial issue.
Alongside the molten rock – magma – inside volcanoes, there is a lesser-known substance: magmatic brine.

Illustration for Ingenia by Benjamin Leon
Another possible option for essential metals is volcanoes. Alongside the molten rock – magma – inside volcanoes, there is a lesser-known substance: magmatic brine. Earth scientists believe that this dense solution of metal salts could address the scarcity of materials.
How brine forms under volcanoes
Many types of brine – highly concentrated saltwater solutions – form in association with volcanic systems, and contain significant amounts of dissolved metal ions, explains volcanologist Professor Stephen Sparks FRS from the University of Bristol. “You can have groundwater leaching through cracks and permeable rock to form saline solutions, for example, and these brines could also be useful,” he says. “But magmatic brine forms from the magma itself.” Solid rock can contain water, such as in hydrated salts or that dissolved into the minerals that comprise the rock. When friction between tectonic plates melts rock to form magma, that water becomes part of a homogeneous molten solution. Through a series of complex geological processes associated with volcanic activity that take place over millions of years, mineral-rich brines accumulate in the sub-surface.
About 10 times as concentrated as seawater, it’s this brine that is of interest for its metallic content. “It tends to occur between the surface and the magma chamber,” Professor Sparks explains. “Many, if not all, active volcanoes are likely to generate brines underneath.” Extinct volcanoes are also likely to have associated brine, as solidifying magma forces water out of the lattice of crystallising minerals.
The liquid brine tends to lie two to four kilometres below the surface in lens-shaped deposits. Engineers routinely reach these depths already in Hawaii and Japan, where they drill to access hot rock for geothermal energy but typically avoid exploring areas where magmatic fluids might be anticipated.
About 10 times as concentrated as seawater, it’s this brine that is of interest for its metallic content. “It tends to occur between the surface and the magma chamber,” Professor Sparks explains. “Many, if not all, active volcanoes are likely to generate brines underneath.”
Magmatic brine already provides some elements. For example, it is extracted and processed to yield bromine in Japan and lithium from the Smackover formation in Arkansas, US. Non-magmatic brine deposits from salar brine (concentrated brine found underneath salt flats, such as those in Chile and Bolivia), already yield lithium. “The extraction of lithium from geothermal brines has been researched for 50 years and for a long time has been technically feasible,” explains Valentin Goldberg, a geothermal energy specialist at the Karlsruhe Institute of Technology. “The problem so far was that, when the cost of lithium is low, conventional resources (such as hard rock mining and salar brine) were cheaper, and thus geothermal lithium was not cost-competitive.”
“These brines can contain any element in the periodic table at concentrations ranging from a few percent by weight down to parts per billion,” says Professor Jonathan Blundy FRS, Royal Society Research Professor in earth sciences at the University of Oxford. “We can find precious metals, base metals, lithium, and even rare earth metals all contained within them. The exact metal endowment depends on the type and location of the parent volcano. This would be a viable alternative to scraping polymetallic nodules off the seabed – and it would do less damage to vulnerable ecosystems.”
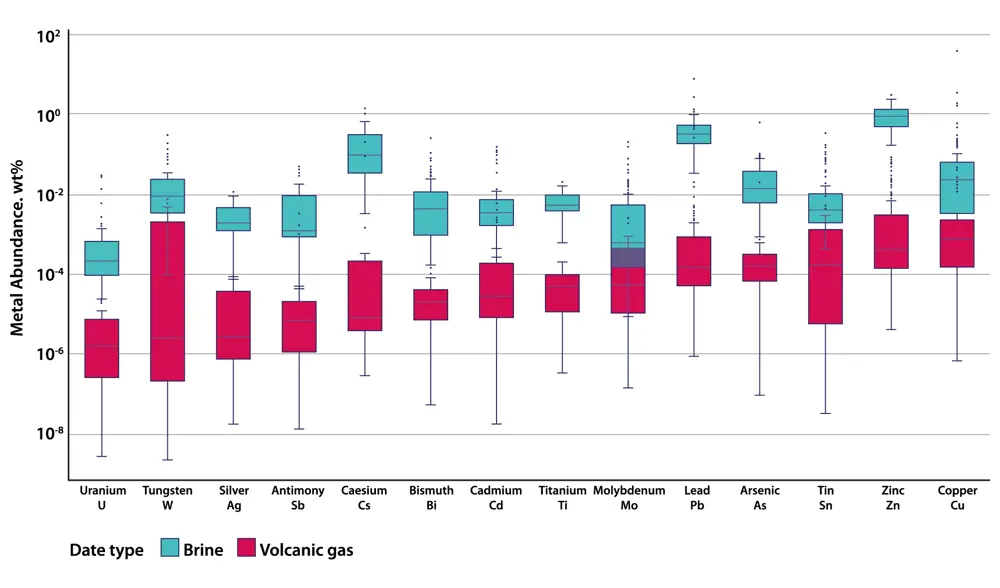
This figure shows compositions of magmatic brines and volcanic gases for representative sodium chloride contents of 50 wt% (weight percent) in brine and 1 wt% in volcanic gas, and listing the metals along the x-axis. Across the broad suite of metals, brines show the most enriched metal signatures, up to three orders of magnitude higher than volcanic gases. Figure adapted from ‘Mining the brine’, by Olivia Hogg and Professor Jon Blundy FRS in Geoscientist, summer 2022
Scientists use magnetotellurics, a process that uses variations of the Earth’s magnetic and electric fields to measure the electrical resistivity of its sub-surface, to locate brines. Brines have high electrical conductivity and can be found using a device similar to a very large metal detector. Professor Sparks explains: “Underneath many volcanoes, like Mount St Helens in the US, you can see regions of very high conductance. The most likely explanation is that there are brines underneath those volcanoes. Unfortunately, rocks saturated in small amounts of brine can still be very conductive. These methods tell you that brine is there, they even tell you the volume of rock which has brine, but they don’t necessarily tell you how much brine is there.”
We can find precious metals, base metals, lithium, and even rare earth metals all contained within [these brines]... This would be a viable alternative to scraping polymetallic nodules off the seabed – and it would do less damage to vulnerable ecosystems.
Professor Jon Blundy FRS, Royal Society Research Professor in Earth sciences, University of Oxford
Professor Blundy explains that determining brine’s composition is a matter of sampling. Scientists employ geochemical modelling to estimate the likely metal content, then use drones to collect samples from volcanic vapours to reconstruct the brine chemistry and deploy geophysics to image the sub-surface.
Goldberg adds: “We can try to estimate the brine chemistry from surface outflows. The fluid chemistry of thermal surface springs can give you already a lot of information about the sub-surface temperature, reservoir type and potential extractable ions.” However, he warns that it isn’t easy to determine whether a particular deposit will be a commercially viable site for mineral extraction as it will depend on flow rate, raw material concentrations and possible extraction technologies.
Other methods to determine the brine’s composition involve analysing deposits found at the surface, says Professor Sparks. “You can look at the minerals that have formed in the brine-forming magmas, which will include small amounts of volatiles and melt, as well as fluid trapped in the crystals as they grow.”
Harvesting and processing metals from magmatic brine
Engineers have several roles in harvesting and processing magmatic brines. They are already working at geothermal energy sites with magmatic brine extraction potential in New Zealand, Japan, Iceland, and Germany’s Rhine Valley. The most obvious role is comparable to oil drilling: operating the drill itself to reach depths of about 4 kilometres and ensuring the integrity of the borehole. “You need to drill into rocks at temperatures around 400°C, and the brines can be aggressive and highly acidic,” Sparks says. “The borehole needs to be lined with a material strong enough to stop it collapsing. Engineers will have to ensure that the lining material can withstand the heat and corrosion by the brine.”
Isabelle Chambefort, Energy Futures Theme Leader with GNS Science, a New Zealand research institute that focuses on geology, geophysics and nuclear science, is investigating brines as an offshoot of work on geothermal energy. She says: “The key challenge in engineering right now is maintaining the well. At high temperature and pressure, you can easily have a collapse of your well at depth.”
One advantage of mining brines rather than seabed nodules or conventional ores is that brines contain fewer impurities. “A benefit is that you don’t have to process rocks to extract metals, as nature’s done that for you,” says Professor Sparks. “So, there are potentially fewer environmental issues associated with mining. Intrinsically, it is easier to extract a brine containing lots of metals, than to mine a lot of rock, and then have to do a lot of chemical engineering and metallurgy to get to the metals back into solution and purify them.”
Engineers have several roles in harvesting and processing magmatic brines. They are already working at geothermal energy sites with magmatic brine extraction potential in New Zealand, Japan, Iceland, and Germany’s Rhine Valley.
However, Professor Blundy adds that “there might still be problems. You might find toxic unwanted metals, such as arsenic or mercury.” He says that we should also consider other issues. For example, there could be induced seismicity, like that associated with fracking, when processed fluids are reinjected into the sub-surface.
Another role for engineering is in processing brines. This could involve the same chemical engineering processes that refine crude oil, which starts with a complex natural material that is processed to give simpler products, in this case purified metal salts. After that, melting the metals might require electrolysis to spur on the chemical reaction. Here, another advantage of drilling into hot rock is likely to come into play, as geothermal energy could provide the electricity needed. Using hot rock as the heating source to generate electricity is well-established. In New Zealand geothermal energy provides 17% of electricity and in Iceland, over a quarter. This could make metal purification self-sufficient and non CO2-generating, says Professor Blundy.
There are several chemical engineering processes under development for extracting lithium from brines. Some of these are already in industrial use, for example with salar brine or residual brine from desalination plants as their starting material. Of particular interest are so-called direct lithium extraction (DLE) processes, which are currently being considered and trialled to extract lithium from geological (non-magmatic) brines in Cornwall. DLE is a multi-stage process: initially it filters the brine to remove any biological contaminants, then concentrates it using selective membranes or ion absorption to remove only lithium compounds from the water. The aqueous residue will be reinjected into the well, and the purified lithium-containing solution further processed using fairly simple inorganic salt chemistry.
A more recent discovery is lithium-ion imprinted membranes – an attempt to mimic biological processes in which ions selectively pass through cell membranes, which is still being studied for its potential to be scaled up for industrial application. In the UK, Imperial College London’s chemical engineering department has a highly active research group looking into selective membrane development.
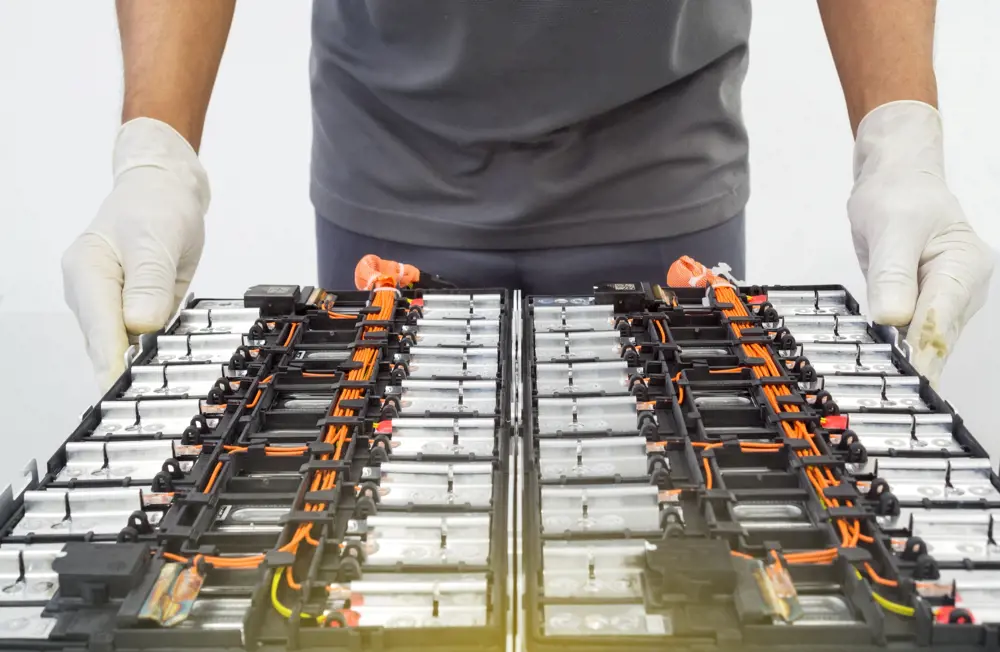
Magmatic brine could be a new source of lithium, which will increasingly be needed for batteries in electric vehicles © Shutterstock
The future for magmatic brine mining
Professor Blundy has been involved in exploratory brine operations, working on drill core samples from Japan, Italy, the Caribbean, and Indonesia. He is part of a consortium of academics from the University of Oxford and its Oxford Martin School working on a project to establish a geothermal power plant on Montserrat, a collaboration with the UK Foreign and Commonwealth Development Office and Department for Energy Security and Net Zero. The team proposes to drill an exploratory well at the island’s active volcano, aiming to reach only the hot rocks above the magma chamber and not the chamber itself. This is the culmination of five years’ work in de-risking the processes needed to extract magmatic brine and will help to quantify the small risk of inducing volcanic activity. Samples already obtained from Montserrat contain small amounts of copper, zinc and lead.
Meanwhile, Goldberg is currently investigating mineral extraction from magmatic brines at geothermal sites in Chile. He believes that continuous production of lithium from magmatic brines will begin before the end of the current decade. The Upper Rhine Graben, also known as the Rhine Rift Valley between Frankfurt and Basel, also has four locations that are promising for lithium and is the target of international projects funded by the German government and the EU.
There is likely to be little magmatic brine in the UK – there are long-extinct volcanoes in Scotland, and may be some brine deposits below the igneous rocks of the bed of the North Sea and near the Hebrides. However, there is, of course, the work in Cornwall looking at extracting lithium from geological brines. Also, several British Overseas Territories, such as Montserrat and Ascension Island host much younger, dormant volcanic systems that hold promise. Regardless of the exact location, UK-based engineers and innovation can play a key role in the technologies used to obtain the metals crucial to a net zero future.
Contributors
Professor Jon Blundy FRS is an igneous petrologist interested in all things magmatic, from magma generation in the crust and mantle to active volcanoes and hydrothermal mineralisation. He has worked on volcanoes around the world including the Eastern Caribbean, Altiplano, Mexico, Cascades, Kamchatka, Vanuatu, Turkey, and Ethiopia. He is a Royal Society Research Professor at the University of Oxford with a project entitled ‘From Volcanoes to Green Mining – Rethinking Igneous Geochemical Cycles’.
Professor Stephen Sparks FRS is Emeritus Professor of Geology at the University of Bristol. His main expertise is in volcanology and in risk assessment of geological hazards. He has contributed to understanding how volcanoes work and the processes that form metal-rich ore deposits from volcanic fluids.
Isabelle Chambefort
Energy Futures Theme Leader with GNS Science
Valentin Goldberg
Geothermal energy specialist at the Karlsruhe Institute of Technology
Stuart Nathan
Author
Keep up-to-date with Ingenia for free
SubscribeRelated content
Materials
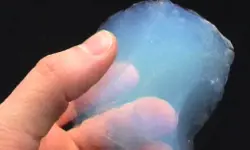
Aerogels
Among some of the lightest known solid materials, aerogels, formed by removing liquid from gels, have many uses ranging from catalysts and sensors, to being used on NASA missions.

Recycling household waste
The percentage of waste recycled in the UK has risen rapidly over the past 20 years, thanks to breakthroughs in the way waste is processed. Find out about what happens to household waste and recent technological developments in the UK.
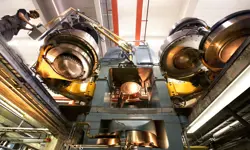
Diamond technology: beyond hardness
Diamond is being used in an increasing variety of industrial and technological applications, due to other properties in addition to its hardness. The UK is home to a wide range of diamond research, development and synthetic diamond production activities.

A lot more than lubrication
The control of friction and wear in mechanical systems by lubrication and surface engineering has led to safer, faster transport as well as medical innovations. Ian Hutchings FREng, GKN Professor of Manufacturing Engineering at the University of Cambridge, highlights the progress and some failures of the important discipline of tribology.
Other content from Ingenia
Quick read
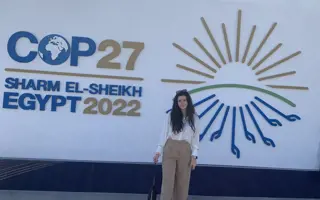
- Environment & sustainability
- Opinion
A young engineer’s perspective on the good, the bad and the ugly of COP27
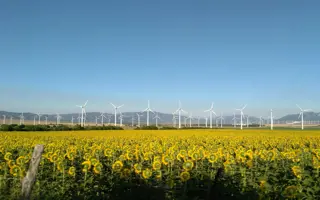
- Environment & sustainability
- Issue 95
How do we pay for net zero technologies?
Quick read
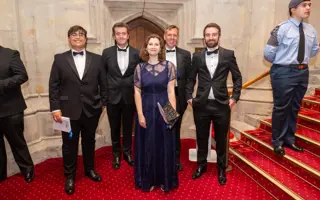
- Transport
- Mechanical
- How I got here
Electrifying trains and STEMAZING outreach
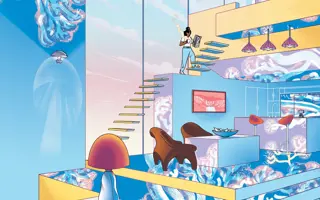
- Civil & structural
- Environment & sustainability
- Issue 95