Mapping the Milky Way
The European Space Agency’s Gaia satellite, dubbed the ‘Rosetta Stone’ of astronomy, is revolutionising our view of the galaxy by charting the position, speed and brightness of more than a billion stars. Ingenia looks at the engineering challenges behind one of Europe’s most ambitious space missions.
Forty-two minutes after its launch on 19 December 2013, the Gaia satellite disengaged from the rocket carrying it and unfurled its sunshield. A controlled thruster burn then set it off towards the Lagrange2 point, an orbit point of high gravitational stability 1.5 million kilometres from Earth, where it arrived safely 20 days later.
The aim of the Gaia mission is to reveal our galaxy in unprecedented 3D detail. From this vantage point, free from atmospheric distortions, Gaia’s telescopes will measure the position of a billion individual stars with a precision of a few microseconds of arc. Over the next five years, rotating on its axis once every six hours, Gaia will map around 1% of our galaxy to produce a vast stellar catalogue, recording the position, motion, brightness, temperature and composition of stars with unparalleled precision.
The European mission had some strong UK engineering input that included the development of light sensors to detect distant stars; the micropropulsion system that keeps these sensors aligned correctly; and the materials specialists who enabled the system to withstand extreme temperatures and minimise vibrations that would have destabilised the telescope.
Professor Gerry Gilmore of the University of Cambridge, the UK Principal Investigator for Gaia, says that for the first time, “we will have a fair sample of what is out there, where it is, how it is moving, how unseen (dark) matter is distributed, where and when stars formed and where and when the chemical elements of which we are made were created.”

The Gaia craft being tested for acoustic stability in a large ESA test chamber in Toulouse, France. It was subjected to a simulation of the acoustic environment it will experience during launch. The extreme acoustic levels during launch are due mainly to the noise of the launcher’s engines, with a smaller contribution from the airflow over the fairing during the climb through Earth’s atmosphere at supersonic speeds © Airbus DS / Dominique Eskenazi
Space challenges
Building bespoke satellites is a long, involved and expensive activity. On this occasion, it demanded innovative solutions with extremely high specifications. The European Space Agency (ESA) approved the Gaia mission in 2001. It employed as prime contractor Airbus Defence and Space (Airbus DS, formerly Astrium). Airbus DS in turn allocated a team of 400 engineers and technicians to design and build the £650 million probe, working alongside 50 manufacturers, 47 of which were based in Europe.
UK industry and institutes won around £65 million of contracts for work on Gaia. This sum included the high‑resolution camera, the control avionics, the micropropulsion system and a role in the development of the Gaia spectrometer.
When conceiving Gaia, ‘ultra‑precision’ was the watchword. The data from Gaia’s three instruments, which take astrometric, photometric and spectroscopic measurements, can pick out objects half a million times fainter than the human eye can see, and will provide pinpoint location for each star mapped with an error margin of only six microarc seconds, equivalent to a penny on the surface of the Moon as viewed from Earth.
To attain this acuity, Airbus DS designed and built two telescopes of extraordinary sensitivity, able to measure the diameter of a human hair – around 17 µm – at a distance of a 1000 km. The telescopes bounce light through a sequence of 10 custom-shaped mirrors, which extend Gaia’s effective focal length to 35m – 10 times greater than the diameter of the payload itself – before focusing it onto the focal plane array.
Mapping the stars

The Milky Way over Weikersheim in southern Germany on a summer’s night © Jens Hackmann
The English astronomer, John Flamsteed, compiled the first stellar catalogue at the Royal Greenwich Observatory in London with the aid of a telescope in 1755, listing the positions of almost 3,000 stars with a precision of 10-20 arc seconds. Several decades later, Jérome Lalande, a French astronomer at the Paris Observatory, published an even greater catalogue of 50,000 stars with a precision of around three arc seconds.
These early stellar maps still lacked one crucial piece of information: the distance to the star, calculated by measuring the apparent movement of a star as the Earth rotates around the Sun (stellar parallax). Once astronomers had mastered that skill, just over a century ago, stellar catalogues from ground-based telescopes rapidly grew in size and precision. However, by the second half of the 20th century, ground-based telescopes began to reach their limit due to distortions of starlight caused by the Earth’s atmosphere.
In 1965, the French astronomer Pierre Lacroute suggested a dedicated space telescope to measure stellar positions. The idea eventually became ESA’s Hipparcos satellite. Operating between 1989 and1993, Hipparcos (an acronym for HIgh Precision PARallax COllecting Satellite that echoes the name of the ancient Greek pioneer of astrometry) charted the position, distance and velocity of almost 120,000 stars with a precision of 0.001 arc seconds. Hipparcos data were used to compile the Millennium Star Atlas, released to the public in 1997, and for planetarium software and other visualisations such as Google Sky. Thanks to advances in technology, ESA’s Gaia probe brings astrometry into the 21st century by charting over one billion stars with a precision of up to 0.00001 arc seconds.
Seeing stars
The focal-plane array is the jewel in Gaia’s crown, a vast digital camera comprised of a mosaic of 106 advanced charge‑coupled device (CCD) light sensors. The CCDs pack in almost a billion pixels into an area of 0.38m² – the largest ever space-bound camera of its kind.
Airbus DS assembled the focal plane at its facility in Toulouse. The UK company e2v designed, manufactured and tested the CCDs at its facility in in Chelmsford, Essex. For e2v, the primary challenge was one of production scale. In 2009, e2v provided two CCDs that were retroactively fitted to a precursor of Gaia, the Hubble telescope. This delivered a tenfold improvement over the previous camera, the Wide Field Planetary Camera 2. By contrast, for Gaia, e2v had to deliver 200 chips over five years, each one the same size or bigger than those attached to Hubble.
Choosing materials
When dealing with equipment of this sensitivity, temperature fluctuations of a fraction of a degree can introduce vibrations or structural expansion, with a catastrophic impact on accuracy. Gaia’s design was therefore guided by the principle of extreme stability, with all components having to contend with the extreme environmental rigours of outer space.
Beyond the development of the CCD image sensors, e2v’s engineers worked with Airbus DS to explore ways to design a broader package that would hold the sensors at the right place, at the right temperature, in a very stable way. Earlier satellites often saw CCDs simply stuck to a metal carrier, mounted and put in place, but the demands of this mission required a new approach.
For Gaia’s mirrors and support structure, Airbus DS trialled materials including carbon-carbon and Zerodur, a low expansion glass ceramic manufactured by the German company Schott AG. In the event, the engineers fixed on silicon carbide, an ultra‑lightweight ceramic material used in earlier craft such as the Herschel Space Observatory. Manufactured for space by the French firm BOOSTEC, silicon carbide has proven itself uncannily suited for space. Twice as rigid as steel, highly durable, and boasting a very high thermal conductivity, the material ensures that Gaia will be highly resistant to expansion or contraction under changes in temperature.
The 3m diameter, quasi‑octagonal torus, built to support the two optimal telescopes and the focal plane assembly, consists of 17 silicon carbide segments, which were brazed into one coherent structure at the BOOSTEC premises at Bazet near Tarbes, France. This structure makes Gaia the largest ceramic space instrument ever flown. Its 710kg scientific payload is held in position to picometre precision.

e2v
Gaia’s digital camera is underpinned by semiconductor imaging technology developed by e2v based in Chelmsford. Founded in 1947, e2v started life as Phoenix Dynamo Company, manufacturing components for radar transmitters. Today, the firm employs 1,600 people across Europe, North America and Asia, and has annual sales of £200 million.
Gaia’s focal plane has 106 CCDs. Technicians from Airbus DS France, the Gaia mission’s prime contractor bolt and align the CCDs onto the support structure at the company’s facility in Toulouse. Made by e2v in Chelmsford, each CCD measures 6×4.7cm, with a thickness of a few tens of microns © Airbus DS
Its high-performance image sensors are used in almost 150 ground- and space-based telescopes, including: IRIS, Gaia, Pleiades, STEREO, SDO, Hubble, Mars Curiosity Rover and Rosetta. However, the combination of Gaia’s large-area CCDs, high‑performance specification and large supply volumes presented e2v with new challenges. The scale of manufacture meant that the facilities needed to be highly automated. e2v developed new test methods during the 12 years that it worked on the project, including an automated routine for testing the modulation transfer function, a standard measure of lens performance.
The team also needed to make sure the CCDs were spaced as closely as possible so as to minimise the dead space between adjacent CCDs in the array. This led to a new wire‑bonding arrangement between the chip and a ribbon flex, which provides the electrical interface to the outside world. e2v supplied three different variants of the CCDs, each optimised for a separate light wavelength and used in different instruments in the array. e2v also subjected the CCDs to their well-established back thinning process, removing bulk silicon to raise peak quantum efficiency (the percentage of light detected) to in excess of 90%.
Other UK firms that have contributed to Gaia, in addition to the UK arm of Airbus DS/EADS, e2v and UCL are: Selex Systems (spacecraft documentation, configuration, schedule support); ABSL (battery technologies); and Aero Stanrew Ltd (avionics model bench structure).
Keeping cool
Gaia’s principal protection from temperature fluctuations is a 10m-diameter sunshield, a circular layer of insulation that sits on the base of the cylindrical body of the spacecraft, like a large-brimmed hat. It is designed to keep Gaia in shadow, maintaining scientific instruments at a constant temperature of around -110°C. The sunshield also supplies power via six rectangular gallium-arsenide solar arrays, with a total surface area of 12.8m², attached to the underside.

Gaia’s deployable sunshield assembly – an array of 12 frames of carbon fibre tubes hinged at the base of the spacecraft – went through extensive testing at Europe’s spaceport in Kourou, French Guiana, in October 2013. In orbit, the sunshield unfurled to create a 10m-diameter sunshade that keeps Gaia in shadow, maintaining scientific instruments at a constant temperature of around -110°C © ESA–M. Pedoussaut
A synchronously deployable thermal shield of this size is a technological first. Its testing and deployment threw up several challenges. With a diameter of 10 m, the shield was too large to fit into the launch-vehicle’s fairing, so it was built from 12 folding panels, designed to unfold over nine minutes once Gaia reached space.
The designers, Spanish engineering group SENER, conceived a design of 12 frames of carbon fibre tubes hinged at the base of the spacecraft, with frames covered with gold multilayer insulation blankets. The device was not designed to support its own weight under Earth’s gravity, so during testing in the clean room at Kourou, ESA’s launch pad in French Guiana, engineers had to rig the panels of the shield to a system of support cables and counterweights to mimic the microgravity of space.
To ensure maximum performance, the surface of the sunshield has to be fully tensed when deployed. Any wrinkles or imperfections can result in a difference in the thermal gradient for a part of the satellite.
The sunshield is one of Gaia’s few moving parts. To minimise vibrations, even the antenna that beams data down to Earth is pointed electronically rather than mechanically. The requirements on the propulsion system for such a sensitive mission are extremely challenging – even simple devices such as valves, or the flow of propellant moving around the chemical propulsion system, can be disruptive.
So, while a chemical propulsion system controlled the spacecraft on release from the launcher and provided orbit corrections during its journey to the mission location, the Gaia team faced the challenge of creating a secondary micropropulsion system to maintain altitude control and keep the telescopes spinning at a constant rate.
The resulting micropropul-sion feed module, manufactured at Astrium Portsmouth, consists of 12 cold‑gas thrusters, grouped in three clusters of four. The thruster system uses high‑pressure nitrogen propellant, controlled by piezoelectric actuators, to provide very small impulses with a thrust of 1 to 500 micronewtons – a force so small that you would need more than a thousand thrusters to support a sheet of paper.

Gaia in brief
The Gaia spacecraft is a hexagonal structure, approximately 3m high and 3.5m in diameter, with a launch mass of two tonnes. It has three main modules: the payload, services and electrical services
Gaia satellite with sunshield unfolded © ESA–C. Carreau
The payload module is built around a toroidal optical bench made from silicon carbide. This provides the structural support for a single, integrated instrument enabling Gaia’s three functions: astrometry, photometry and spectrometry. It also contains the electronics for managing the instrument’s operation and processing its raw data.
The service module comprises all mechanical, structural and thermal elements supporting the instrument and the spacecraft electronics. It includes the micropropulsion system, deployable sunshield, payload thermal tent, solar arrays and harness. The electrical service module provides support for the Gaia payload and spacecraft for orientation, electrical power control and distribution, central data management and radio communications with the Earth.
The majority of e2v’s 106 CCD sensors are devoted to Gaia’s astrometry instrument, with its two large primary mirrors. By determining the angle between stars as the craft revolves, this instrument can determine how far away a star is via parallax – the difference in the apparent position of an object viewed along two different lines of sight.
The remaining CCDs, located on the same silicon carbide support, serve Gaia’s two other main instruments: a photometer made from two fused-silica prisms that disperse the light, offering a measure of a star’s spectral energy distribution, from which its temperature can be inferred; and the radial velocity spectrometer (RVS), which records the spectral signature of a star to determine its speed of travel in the line of sight and provide more detailed astrophysical measurements such as a star’s metallicity – the proportion of its matter made up by elements other than hydrogen and helium.
Preparing for liftoff
Silicon carbide is perfect space material, but it is also brittle, and getting a satellite as fragile as Gaia beyond earth’s atmosphere raised serious challenges. During its launch on a Soyuz rocket from Europe’s spaceport in Kourou, Gaia’s scientific payload was attached to the service module by three heavy‑duty struts, designed to carry the mechanical and thermal shocks. Once the craft was outside Earth’s gravity, the struts were broken by command to leave the payload supported by much thinner rods made from composite materials that minimise thermal or mechanical conductive disturbances from the service module.

Supported by the launch vehicle adapter (yellow), the Gaia spacecraft (orange) is positioned inside the Soyuz fairing (white) in preparation for launch © ESA–M. Pedoussaut
With Gaia operating 1.5million kilometres from Earth, the engineers had to get it right first time. In November 2013, ESA postponed Gaia’s imminent launch after it identified a technical issue in another satellite that shared components used in Gaia’s transponders, generating ‘timing signals’ for downlinking the science telemetry.
Phoning home
Dealing with the vast amount of data that Gaia harvests posed another challenge for Airbus DS. The CCD array will collect one billion pixels’ worth of data every few seconds, a total of 73,000 terabytes over the project’s five-year lifespan. Transmitting this enormous quantity of data back to earth created numerous challenges, with factors to consider including cost, mass, power consumption and reliability considerations.
To get around this, Airbus DS engineers in the UK developed a first level of on-board processing, powered by a series of seven parallel video processing units, each exhibiting a processing power of more than 1,000 MIPS (Million Instructions Per Second). These compress the data in a similar way as computer compresses files, cutting out elements of data (the gaps between points of interest) and reducing Gaia’s data rate by a factor of 300.
Gaia sends this data back to Earth at a rate of around 10 million bits per second using a phased-array high-gain antenna. Electronic beam steering allows the signal to be directed towards Earth as the spacecraft rotates, and eradicates microvibrations that might impact the performance of the telescope.
Data beamed back will be in a raw form that needs to be processed in order to create a calibrated set of measurements that the astronomical community can freely use. Even in a reduced form, the scale of the data is so huge – an approximate one petabyte, or one million gigabytes, over five years – that ESA has established a pan‑European data processing and analysis consortium, involving 450 people spread across academic institutes and space agencies.
Looking forward
After the initial pause, the launch of the Gaia probe went without a hitch. Its data-crunching teams expect to start issuing its first results in 2016, with a full billion‑star catalogue due in 2022.
Meanwhile, the technological innovations that made Gaia possible will influence many other areas in years to come. For example, e2v reports high demand for its CCD technology for use in ground‑based telescopes, forthcoming space projects, and other high‑accuracy detection systems such as industrial machine‑vision cameras. Gaia’s telescope mirrors offer a model for the optical technologies, while silicon carbide can be a stable and lightweight alternative to monolithic glass, beryllium and aluminium in structures for space, aerospace and telescopes.
The cold-gas micropropul-sion system used to maintain Gaia’s orientation and position outlines a high‑performance propulsion principle for future state‑of‑the‑art space missions. Gaia’s phased-array antenna offers a future model for data transmission over extreme distance.
The launch of Gaia marks the end of an era for more than 1,000 engineers, scientists and technicians who worked on the project since its approval in 2001. Professor Mark Cropper, Deputy Head of UCL’s Mullard Space Science Laboratory, who helped develop the radial velocity spectrometer, described the launch as “A mixture of worry, pride, relief and sadness. Scientists wanted X, engineers wanted Y, and funding agencies said the budget is Z, so it all had to go into the pot and then, 15 years later, Gaia is the amazing result. It takes the coming together of many different people to make it happen and for many this ends now.”
The lessons learned in the design, construction and launch of Gaia have already influenced future space exploration. Many of the UK teams involved have gone on to apply their experience to upcoming space science projects, including Euclid (launching in 2020) and Plato (launching in 2024). Future missions will exploit the techniques developed for Gaia to speed up data processing, keep satellites cool and hold delicate equipment steady and in place.
Matthew Chalmers and Louis Pattison would like to thank Professor Mark Cropper, Deputy Head of UCL’s Mullard Space Science Laboratory; Peter Bennie, Project Manager for Gaia’s video processing unit for Airbus DS; and Paul Jorden, Technical Specialist at e2v for their help in writing this article.
Keep up-to-date with Ingenia for free
SubscribeOther content from Ingenia
Quick read
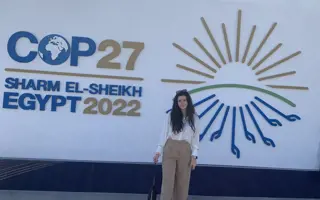
- Environment & sustainability
- Opinion
A young engineer’s perspective on the good, the bad and the ugly of COP27
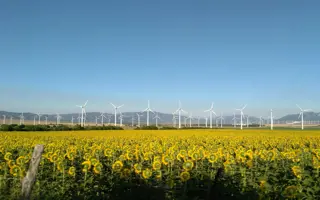
- Environment & sustainability
- Issue 95
How do we pay for net zero technologies?
Quick read
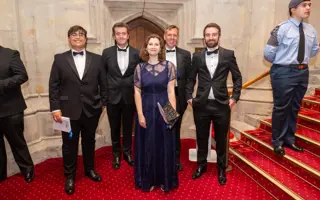
- Transport
- Mechanical
- How I got here
Electrifying trains and STEMAZING outreach
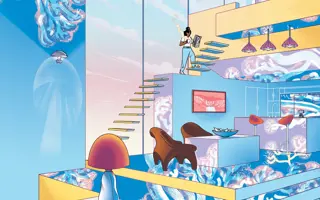
- Civil & structural
- Environment & sustainability
- Issue 95