Larger, further and faster: Passenger planes of the futureDeveloping larger aircraft: The engineering challenges of the Airbus A380
The development of new aircraft takes place over considerable time scales, so aircraft designers need to make realistic assessment of future needs. Early decisions, once made, will impose their own limitations on many aspects of a new aircraft design. This article describes the thinking behind the development of the Airbus A380.
The symbols of twentieth century air travel have arguably been Concorde for speed and the Boeing 747 for mass intercontinental travel. Notwithstanding the horrific events of 11 September 2001, it is still anticipated that in the long term air traffic will grow by up to 5% per annum, leading to twice the year 2000 volume by around 2015 and treble by 2025. What are the driving forces in the development of the flagship aircraft of the 21st century?
Speed has always had its attractions, but perhaps less so now in the context of today’s environmental awareness, with the fundamental laws of physics telling us that the fuel burnt per passenger per kilometre increases with speed. The predominant drivers will be to allow those people who wish to travel to do so at affordable fares, reliably, on time, and with adequate comfort (and health). This will need to be with minimal environmental impact, either at airports, or with emissions and noise affecting the general nontravelling population. Obviously aircraft developed with these criteria in mind will also need to support the business objectives of the airlines and the manufacturers.
The extension of the Airbus family
The Airbus response to these driving forces is the development of the A380, the company’s new flagship for the 21st century. This extension to the Airbus family (Figure 1) is designed to give airlines greater choice and maintain existing economies such as ‘cross crew qualification’. The ‘common cockpit’ concept has already given the airlines savings by combining with ‘flyby- wire’ capabilities to minimise differences in control characteristics and hence reduce additional crew training in and between the various Airbus families of passenger aircraft. The A380 will extend this concept while providing an aircraft with a capacity of around 550 passengers in a three-class layout.
As traffic increases, airlines will be able to develop more point-to-point routes, or ‘trade up’ for extra capacity to larger aircraft. A general increase in the size of aircraft is inevitable if traffic growth is to continue without being throttled by take-off and landing slot availability and further overcrowding of the skies.
The ‘large aircraft’ solution
So, given the requirement, what is the solution? Under the original A3XX label, several configurations were looked at by Airbus to satisfy the ‘large aircraft’ requirement, particularly to stay within the ‘80 metre box’. This is the dimensional limit agreed with the leading airport authorities to keep any necessary infrastructure development of existing airports to accommodate new aircraft within reasonable economic bounds. Among other configurations, a ‘flat figure eight’ double bubble fuselage and a three-surface foreplane–wing– tailplane layout were looked at. However, in the end, the studies homed in on a twin deck conventional configuration that developed into the A380 project launched in December 2000.
One of the most crucial decisions taken was the fuselage cross-section (Figure 3). This had to offer twin-aisle flexibility on both decks with increased comfort standards for all 555 passengers and also to ensure adequate dimensions for cargo containers on three decks to give 150 tonne plus capacity. The bottom deck also has to have adequate ‘standing’ height to be used for additional passenger facilities, such as bars, casinos or crew rest areas, although we are unlikely to see too many jacuzzis, as appeared in some early artists’ impressions! It was also important to ensure that at least a first potential fuselage stretch to give 656 passengers could be accommodated within the 80 m length limitation.
The final choice of cross-section then drove many other aspects of the configuration. Relatively speaking, the fuselage is, for example, quite short compared with the dimensions of the wing. Much development was then required of the emergency evacuation configuration: the emergency exit size and positioning, and escape slide development for the upper deck and away from the wing. Another aspect was ensuring quick turn-around capability, which required normal passenger access to each deck simultaneously with adequate space for the ground handling equipment, baggage and cargo loaders, catering trucks, etc. This then constrained the inner wing dimensions and engine intake fore and aft positions to some extent, requiring total integration of the configuration development at the aircraft level. Another infrastructure constraint was the ‘pavement loading’ or bearing strength of the runways, aprons, etc. While a few taxiway bridges will need to be strengthened because of the absolute weight of the aircraft (up to 620 tonnes in developed versions), the landing gear arrangement ensures that no general strengthening of runways, taxiways and terminal apron areas will be required. This has necessitated the use of a 20-wheel main gear arrangement to spread the load across two 4-wheel wing mounted bogies and two 6-wheel fuselage mounted bogies, with the rear axle of the latter being steerable.
The engineering challenges
As well as the detailed complexities introduced by the configuration of the aircraft, in spite of its ‘traditional’ layout, the challenge facing the design and production teams was, and is, huge. It is the sheer size of the aircraft which is driving many, in fact most, of the technological innovations on this project.
Long before the advent of the A380, text books often stated that aircraft size would be limited by the ‘square-cube law’. That is, as size increases, the area for passengers and the lifting capability of the wing (for example) increase as the square of the linear dimension; however (at constant material properties), the weight increases as the cube. The normal economies of scale would thus eventually be overtaken. In the past this limitation has been overcome by continual technological improvements in aerodynamics, material properties and engine efficiencies. However, the science of subsonic flight is maturing and the Airbus design team and the engine suppliers have had to work very hard to continue the historical trend towards improving the economics when carrying so many passengers in one air vehicle.
To an extent the A380 fuselage has cheated the ‘square-cube law’ by incorporating two passenger decks. Compared with using one deck, this provides a step change in the efficient use of the volumetric space. However, with the wing, for example, the law has most certainly made itself felt in the parameters controlling the dimensioning of the main structural load-carrying wing box. This has further intensified the usual drive towards weight reduction over the whole of the aircraft.
A different challenge was the need to give airlines the flexibility to operate very long range flights once they had committed to a major investment in a new aircraft. This was particularly so because much of the anticipated long term traffic growth is expected to be within the Far East, and to and from Europe, the USA and the Far East. The capability to carry the design payload over routes such as Singapore–London under all adverse wind conditions then became essential. This required some increase in speed and Mach number (to M = 0.85–0.86) compared with earlier Airbus projects. At the same time it was necessary to minimise transonic aerodynamic drag which, with aircraft weight and engine thermodynamic efficiency, determines the fuel burn and hence fuel load carried.
With the sophisticated computational fluid dynamic methods now available, at cruise conditions the advanced wing is designed to carry supersonic flow over more than half of its area, but terminated by such weak shock waves that drag from this source is less than 2% of total aircraft drag. The flow pattern is very complex and highly three-dimensional, being affected by fuselage and engine nacelle influences over most of the span.
The aerodynamic aspects of development also interacted strongly with the weight reduction campaign: much work was done to optimise the wing planform in sweep and taper, and thickness-chord ratios, to ensure the best balance between wing weight and wing drag for this size of aircraft. This resulted in a wing loading distribution across the span with a more inboard centre of lift than the ‘elliptic loading’ often thought of as giving the minimum induced, or vortex, drag which is fundamental to the generation of lift. This then implied an optimum span for the A380 somewhat larger than the 80 m limit, which resulted in exchanging some span for a vertical surface at the wing tip, effectively giving a similar aerodynamic benefit to the higher span (Figure 5).
At low speed, the emphasis on the high lift device’s design once again was one of simplicity, low drag and light weight while maintaining adequate take-off and landing speeds. This time, ‘low drag’ was to a large extent driven by airport noise requirements, particularly the ‘QC2’ standards which would allow more flexible operations out of London Heathrow Airport. Current airport noise requirements have a decibel limit which increases with weight but with an absolute cut-off (at about the weight of the Boeing 747!). The limit therefore becomes more of an issue for the very large aircraft. This has significantly affected the high lift device design as well as the engine and nacelles, particularly for take-off conditions, leading to changing from the traditional Airbus slotted slat to a non-vented ‘droop nose’ arrangement on the leading edge inboard of the inner engine.
Returning to the weight challenge, although these are not obvious to the casual observer, the A380 will incorporate many technological improvements. These range from using the fly-by-wire computer flight control laws to minimise flight loads in normal cruise, and in manoeuvres and turbulence, through new materials and production techniques to new structural and system concepts. As well as enabling the ‘common cockpit’ concept the Airbus computerised fly-by-wire flight control and flight envelope protection systems are now well proven on the A320 and A330/A340 families of aircraft and well liked by pilots. A further step has been taken on the A380 towards a fully integrated ‘modular’ avionics system, which starts to use common computer modules controlling different sub-systems of the aircraft and able to take over functionality if other modules fail. Following the pattern adopted on the A340-600, going into service in mid- 2002, the A380 has no mechanical reversion on any flight control system – it is a 100% fly-by-wire aircraft.
As well as allowing new and traditional control law enhancements to improve passenger comfort and reduce design loads, the flying control system architecture also allows for increased redundancy and safety standards. For the first time on an Airbus aircraft, one of the usual three hydraulic systems has been replaced by an electric power distribution system driving ‘electro hydraulic actuators’ on the aerodynamic control surfaces. These are self-contained closed circuit electric pumps driving a hydraulic ram actuator, with both the position signalling and power supplied electrically. Some of the actuators also have the capability of being supplied by a hydraulic supply as a back-up if the electric power supply fails. The overall architecture then gives effectively four independent flight control systems on the aircraft, but still with a significant weight saving compared with three hydraulic systems, through the use of new lightweight aluminium power cables. The supply pressure in the hydraulic systems has been raised from the normal 3000 psi to 5000 psi for the first time on a civil aircraft (overtaking Concorde, which uses 4000 psi for its hydraulic systems), reducing fluid volumes and pipe sizes again to reduce weight, and to avoid sealing challenges with the very large diameter pipes otherwise needed.
There are also many innovations in the design of the structure of the aircraft, every aspect of which is being intensively investigated to ensure the most cost effective lightweight design is applied, be that through the use of advanced materials, new manufacturing processes, design concepts, control of loads or just good basic design of all details down to the last bracket. All technology selections made for the aircraft involve a review of the effects over the whole life of the aircraft: operation, maintenance and repair aspects are all considered. Any new technology must finally provide real benefit to the airlines and passengers. Some of the structural developments are indicated in Figure 4.
Airbus has been at the forefront of introducing carbon fibre reinforced plastics into primary as well as secondary structures on large civil aircraft. With the A380 a further step has been taken by introducing a CRFP wing centre section as well as the nowtraditional Airbus carbon fin and tailplane. The size of these carbon structures is huge: the tailplane is as large as the wing of the A310 at 205 m2 area and a person can quite easily stand up in the 7.1 × 6.6 × 2.4 metre wing centre box section.
A contribution from the UK
As for all previous Airbus aircraft, the main wing structure is the responsibility of what is now ‘Airbus UK’ in the new integrated company, with design and some manufacturing at Filton, near Bristol and final assembly at an all-new facility at the Broughton plant in North Wales, near Chester. Again, the challenges are driven by size, with each half wing being 45 metres long from root to tip and, with its transportation vehicle, weighing 125 tonnes. The wing sections will be transported by barge on the nearby River Dee to Mostyn Docks and then by sea to Bordeaux and onwards by road to final assembly at Toulouse.
In the early design phase various combinations of metal inner wing and outer carbon wing were investigated, but, given that a complete carbon wing on such a huge scale was a step too far, then the cost and weight penalty for a major mid-span metal/carbon joint could not be overcome and the wing has stayed with mainly metal construction. However, aluminium technology is being pushed to its limits, with new alloys being specified which are still going through their qualification process. The size of the components is also driving a new look at the traditional sizing criteria, with major wing ribs probably moving to a carbon design in what is basically a metal wing. In contrast, with smaller wings with carbon covers the ribs often remain metallic, since this provides the most cost to weight effective solution.
A global project
This is a project of global proportions and although it is led by Airbus and its operating companies in France, Germany, Spain and the United Kingdom, European companies from almost all the EU member states are involved. Major airframe, equipment and component suppliers from the United States, Canada and Japan will also participate. On the engine side, two companies from the UK (Rolls Royce) and the US (Engine Alliance) are preparing to supply the A380 with advanced technology engines, both involving world-wide supply chains. The scheming and detailed design for component manufacture are well under way, looking towards final assembly of the first aircraft starting in 2004. The first flight of the A380 is targetted for Spring 2005 with entry into service planned for 2006.
Jeff Jupp recently retired as Director Technical, Airbus UK, and is currently Visiting Professor at Bath University. Having graduated with first class honours at Queens’ College Cambridge, he started his career in Civil Aircraft Design in the Aerodynamics Department at Hawker Siddeley, Hatfield, before moving with the Airbus project to British Aerospace at Filton, Bristol, where he was appointed Engineering Director in 1993. He is the joint holder of the 1993 Royal Society ‘Esso Energy Award’ Gold Medal.
Keep up-to-date with Ingenia for free
SubscribeOther content from Ingenia
Quick read
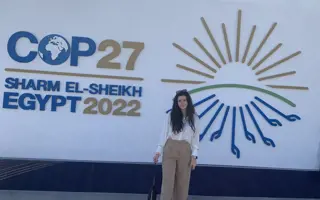
- Environment & sustainability
- Opinion
A young engineer’s perspective on the good, the bad and the ugly of COP27
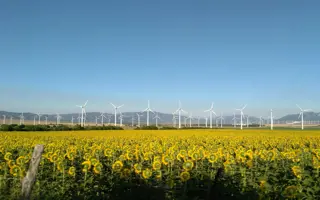
- Environment & sustainability
- Issue 95
How do we pay for net zero technologies?
Quick read
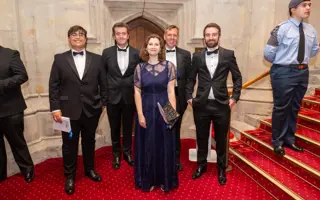
- Transport
- Mechanical
- How I got here
Electrifying trains and STEMAZING outreach
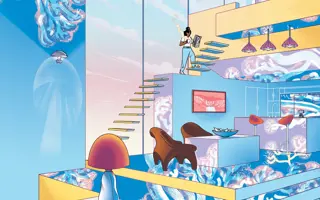
- Civil & structural
- Environment & sustainability
- Issue 95