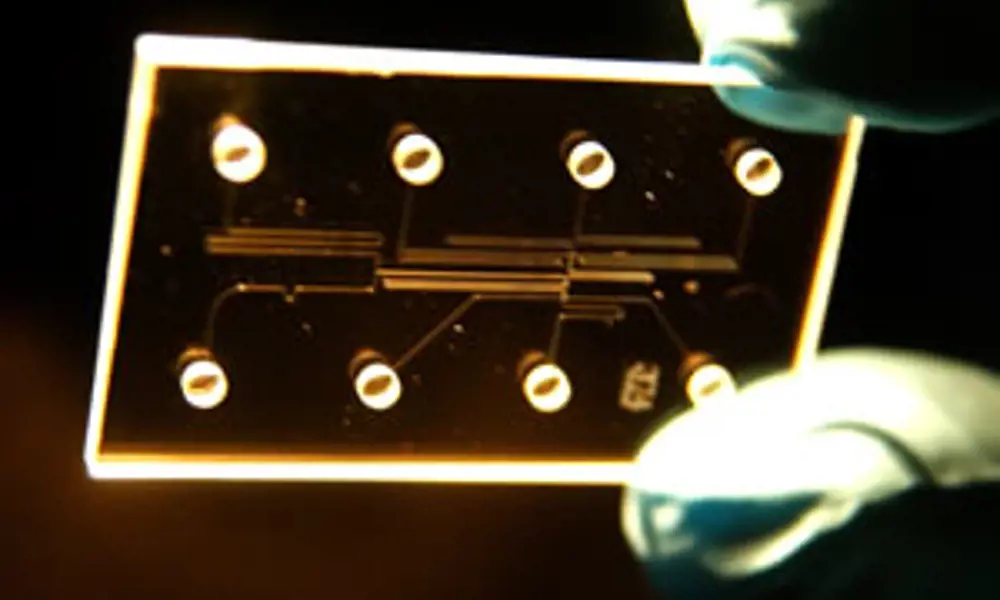
Lab-on-a-Chip
The eight holes on this chip are actually ports that can be filled with fluids or chemicals. Minituarised valves control the chemical processes by mixing fluids that move in the microchannels that look like lines, connecting the ports. NASA scientists designed this chip to grow biological crystals on the International Space Station © NASA
Lab-on-a-chip technology has revolutionised medical diagnostics. It has also been used to help us to understand the human genome and is poised to search for extra-terrestrial life. Professor Jon Cooper FREng, a leading exponent in the field, writes about the rapid development of this form of analysis.
In 1979, American researchers based at Stanford University unveiled a miniature gas analyser that would change the face of chemical analysis. Borrowing standard chip-making techniques from the microelectronics world, Steve Terry and colleagues built a gas chromatograph on a single silicon wafer that could separate and detect gases from a mixture, in seconds. It was one of the first examples of a lab-on-a-chip.
Ten years later, researchers were busy fabricating the first pumps and sensors to make other types of micro-sized tools to investigate analytical reactions using minute samples. Today, scientists and engineers worldwide are building entire microsystems that automatically perform all stages of chemical and biological analysis, from sample preparation to data analysis, be it for detecting disease in the Developing World or looking for life on Mars.
CHIPS OF CHOICE
The typical lab-on-a-chip, also known as a microfluidic chip, is usually a micromachined glass or plastic substrate that contains a network of channels only a few tens of micrometres in diameter, within which sensors and other analytical devices are located. These channels can be used to manipulate fluids precisely thanks to the well understood phenomena associated with flow and diffusion at the microscale.
Although silicon is the micro electronics industry’s material of choice, glass or plastic substrates are commonly used for optical measurements as they are not only cheaper but have the important property of being transparent. The use of microfabrication and micromachining protocols, adapted from the semi-conductor industry, enables a range of different analytical methods involving microsensors, pumps and separators to be integrated onto the chip, thereby shrinking and streamlining all laboratory techniques onto it. Indeed, when several analytical protocols are implemented into a single chip, the result can be provided within seconds (a technological solution that takes analysis all the way from “sample to answer”).
THE IMPORTANCE OS SIZE?
As with many technologies, cost and speed are the bottom line; a look at the electronics industry provides an explanation. Over the past 40 years, two of the major drivers have been miniaturisation and integration. Fabrication techniques have enabled electronic components to become smaller, so that their sizes are now often measured in 10 s or 100 s of nanometres. As a consequence, today’s microchips are cheaper and can process data at much faster rates than ever before.
These trends towards faster and cheaper devices also exist in the diagnostics, biotechnology, chemical and pharmaceutical industries. Low costs are crucial and the need to process and analyse samples quickly – albeit, chemical or biological information, rather than electronic data – is imperative. So it is not surprising that miniaturisation and integration are both now becoming equally important in the life sciences.
When a biological sample and reagents are added to a reaction vessel for analysis, be it a test tube or a lab-on-a-chip, many reactions may take place. In order to react, molecules move towards each other, a process known as mass transfer. In diffusion controlled reactions (where the fluid flow is stationary), an ‘equilibrium’ will be reached over a period of time. Decreasing the distance over which molecules have to diffuse by reducing the size of the reaction vessel, decreases the time taken for this equilibrium to be established (by the square of the size reduction). Making a vessel 100 times smaller (a reduction in size from a centimetre to 100 microns) will mean the equilibrium is reached 10,000 times faster, leading to a faster result.
Indeed, when biological samples are added to the microsized lab-on-a-chip, the reactants reach equilibrium in a few hundreds of milliseconds. The same reaction in a test tube could take many minutes, or even hours. Finally, smaller sizes mean lower costs. Miniaturised devices occupy less space within an instrument and cost less to make, transport, and store. Importantly, smaller devices also consume less reagents, for example, synthesised DNA, used on a biosensor chip, can cost up to £1 million a gram.
CHEAPER CHIPS
A number of microfabrication techniques are currently used to create lab-on-a-chip devices. Laboratory researchers typically turn to the standard photolithography processes used in microelectronic chip manufacturing to make their lab-on-a-chip. Here, light is used to transfer geometric shapes, patterned on a mask, into the surface of a polymer or glass substrate.
While this widely used fabrication route has resulted in a vast number of novel devices, it is generally too costly to be used in the commercial manufacture of lab-on-a-chip devices. However, cheaper alternatives are emerging. Examples of this are the standard moulding techniques used to produce polymer components, which have been successfully applied to plastic lab-on-a-chip devices. Here, moulds of various designs can be used as masters to produce multiple copies of the desired network of fluidic channels in plastic substrates. Meanwhile, glass-based chips, although more difficult to manufacture, can be fabricated when necessary by chemically etching channels into a glass block. In the most recent developments by George Whitesides at Harvard University we see paper being used as the microfluidic substrate with flexible electronic displays printed on them.
These techniques herald the onset of lab-on-a-chip manufacture on a mass scale. Indeed, the Department for Business, Enterprise and Regulatory Reform is currently funding the creation of several lab-on-a-chip manufacturing centres around the UK, based upon both polymer and glass chips.
As demand for smaller, faster and more convenient diagnostic techniques increases, medical industry heavyweights in the diagnostics and pharmaceutical sectors are developing much more robust techniques for making lab-on-a-chip devices. With mass production of these devices on the horizon, where can we expect to see lab-on-a-chip devices being used?
HEALTHCARE USES
Chip-based technologies are already helping to deliver faster and cheaper ‘quantitative biology’. Take the DNA chip, or ‘gene chip’, consisting of arrays of DNA strands attached to a glass or plastic substrate. The DNA segments attached to the substrate act as probes and can detect DNA in a test sample. The chips can then identify mutations or changes in a gene which may be a marker for a given disease. For example, such chips have already been used to study which genes are activated during disease or which genes are turned on or off by new candidate medicines, in the drug discovery process, within the pharmaceutical industry.
Lab-on-a-chip technologies are also being used in DNA amplification. This is the process of repeatedly copying a piece of DNA to increase the amount available for diagnosis. The technique is widely used by forensic scientists and biomedical researchers. The chips are ideally suited to handling the very small amounts of material associated with such DNA diagnostics and have already been integrated into handheld devices for rapid testing in the field.
The amplification of the DNA involves a thermal cycling process – heating and cooling the sample. The small size of a chip, with its low thermal mass, enables this cycling process to be implemented much more rapidly. Such tests may be of equal value in homeland security and in disease diagnosis (in the identification of bacteria).
These are just two examples of how lab-on-a-chip devices are already being used to improve a variety of diagnoses. As the technology evolves, more and more low cost medical diagnostic devices are being developed for use in new applications. Examples extend beyond DNA analysis and include new medical diagnostics for cancer and heart disease – see box overleaf.
DEVELOPING WORLD DIAGNOSTICS
In remote regions of Africa, Asia, and South America, infectious disease remains a main cause of death and creates poverty through its legacy of both mortality and morbidity. Diagnosing disease is difficult under such conditions. The reality of using and maintaining sophisticated analytical equipment, routinely, in the field almost impossible.
However, since the late ‘80s, the use of lab-on-a-chip devices in remote settings has been widely heralded as one of the most powerful potential applications of the technology. In such an environment, a diagnostic tool must be portable and robust, able to perform sensitive analyses swiftly, require minimal user intervention and servicing, and run on very little power. Clearly the lab-on-a-chip, with its small size, low-volume requirement for samples and rapid analysis, fits the bill. Thankfully, philanthropic foundations, such as those established by Doris Duke as well as Bill and Melinda Gates, share this vision.
Only in April of this year, the Bill and Melinda Gates Foundation announced round three of its so-called Grand Challenges Explorations. This five-year, $100 million initiative encourages bold and unconventional research into global health and welcomes proposals on low-cost diagnostics.
Funding such as this has brought about rapid progress in the integration of analytical methods into a single package. Indeed, a wealth of portable and cheap devices are now being developed that perform complex analyses ‘in the field’.
LAB-ON-A-CHIP: bSURE BOWEL DIAGNOSIS DEVICE
Spun-out from the University of Glasgow’s Department of Electronics, Mode Diagnostics is developing a range of diagnostic devices for use in the home. Using electrochemical biosensors based upon new intellectual property, these devices are expected to meet a growing demand for personal health information and point-of-care testing at home.
The bSURE testing device combines lab-on-a-chip biosensor technology with microfabrication and electronics to allow individuals to test themselves for signs of bowel cancer. Bowel cancer is the third most common form of cancer in the UK but if it is detected early enough, it is usually treatable. The bSURE device will be able to rapidly detect occult blood in stool samples and deliver a quick result to help inform the user what action to take.
The device is composed of three parts: the reader with a digital display, a ‘plug and play’ cassette that contains the biosensor and a sampler. When a faecal sample is presented to the sensor by the microfluidic sampler, bSURE detects haemoglobin and gives a digital readout. There is a proportional relationship between the amount of haemoglobin present in the sample and the signal. For the consumer market, the device will report a positive or negative result, as an electronic readout. Devices aimed at the professional care market will be able to report quantities of blood present in a sample.
Future plans include biosensor cartridges to detect signs of infectious disease, other cancers and infertility.
NOT-SO LONELY PLANET
Looking beyond the Developing World, lab-on-a-chip technologies have also found their way into space. The devices have already been used to investigate the effects of micro-gravity on cell growth, detect microbes and contaminants in spacecraft and monitor crew health. However, technologies are now under development that could be used in the search for life on other planets, or indeed to monitor the health of astronauts on extended trips (for example, a prolonged stay in space induces diabetes in astronauts).
In the context of the search for life in space, optical techniques such as Raman spectroscopy – commonly used in chemistry to identify molecules – have been adapted, miniaturised, and integrated in lab-on-a-chip devices. Our research team at the University of Glasgow, working with colleagues at the University of Aberdeen, have already tested such a device within the harsh environmental conditions of the Arctic Circle and initial work has revealed that low-cost instrumentation can identify biochemical markers in micro-organisms within rock samples.
These signatures for life in extreme environments could exist on other planets and as a result a number of robust and ultra-sensitive devices are now being developed for use in the search for extra-terrestrial life. Indeed, NASA is currently funding several research projects that could detect life on Mars. For example, a team of Harvard Medical School and Massachusetts Institute of Technology researchers is currently developing a device to sequence DNA from organisms on Mars. Using recent advances in microfluidics and embedded systems, the team aims to build an instrument that will isolate, amplify, detect, and classify any DNA-based organism from soil, ice or brine samples. A lab-on-a-chip, integrated into the instrument, will detect the DNA and the team hopes to make the instrument small enough to be accepted onto NASA’s Mars mission in 2018.
NASA is also supporting research at the University of California, San Diego, to develop an instrument based on a lab-on-a-chip, that will look for signs of chirality in amino acids, as an indicator of life. This instrument is scheduled to travel to Mars on the European Space Agency’s ExoMars mission planned for 2016.

Astronaut Sandra Magnus works with the lab-on-a-chip portable test system in the International Space Station © NASA
FUTURE DIRECTION
In addition to Developing World diagnostics and Space applications, other fields are rapidly emerging. Synthetic biology is one such field, being the design and manufacture of biologically-based devices and systems. The Royal Academy of Engineering have published a report on this field in May 2009 entitled Synthetic Biology: scope, applications and implications.
In a second emerging area, single cell biology on-chip, which allows researchers to isolate and observe single cells, is becoming an important tool for drug discovery in the pharmaceutical industry. More exciting developments will take place in the world of medicine. Further integration and miniaturisation of lab-on-a-chip devices is probable, enabling in vivo diagnostics in the gut or blood. Indeed, trials have already taken place in our group at the University of Glasgow to test a diagnostic device that is swallowed by a patient to collect data as it passes through their intestine.
Whilst this so-called lab-on-a-pill has been under development for several years, recent advances in sensor integration and data processing have brought exciting results. An array of sensors have been fabricated to perform a range of physiological tests simultaneously. Through efficient use of battery power, the lifetime of the ‘pill’ has been extended. Trials have shown that wireless communications can reliably transmit data, while the addition of a magnet has enabled the sensing device to be tracked in-situ.
These are exciting times for lab-on-a-chip technologies and we can expect many more innovations in the coming years. One major development is the possibility that several sensors could be distributed within the body to improve the quantity and quality of medical diagnostic information, thereby boosting our understanding of the pathology of relatively inaccessible organs.
Further reference
University of Glasgow Bioelectronics Research Centre,
www.gla.ac.uk/electronics/research/bioelectronics
BIOGRAPHY – Professor Jon Cooper FREng
Professor Jon Cooper holds the Wolfson Chair in Bioengineering. He has developed a range of technologies associated with lab-on-a-chip for diagnostics, cell measurements, and proteomics. The primary focus of his work is the demonstration of analytical advantages of studying biological systems at the micro and nanoscale. He has also been closely involved in the commercialisation of chip-based diagnostic devices for the detection of bowel cancer – see www.modedx.com
Keep up-to-date with Ingenia for free
SubscribeOther content from Ingenia
Quick read
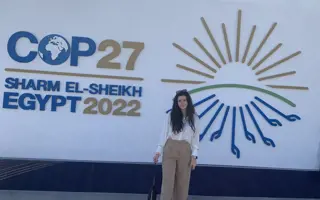
- Environment & sustainability
- Opinion
A young engineer’s perspective on the good, the bad and the ugly of COP27
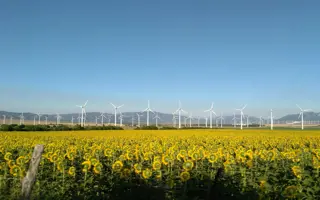
- Environment & sustainability
- Issue 95
How do we pay for net zero technologies?
Quick read
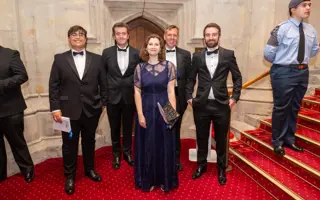
- Transport
- Mechanical
- How I got here
Electrifying trains and STEMAZING outreach
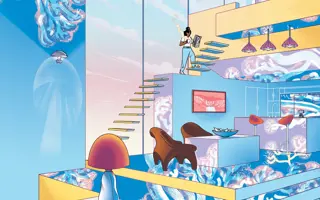
- Civil & structural
- Environment & sustainability
- Issue 95