
Going Underground
The Alpine House at Kew gardens opened in June 2006 Andrew © McRobb, RBG Kew
For many years the potential of using the earth as an energy resource for buildings has been overlooked. Patrick Bellew FREng is one of a small number of people who has been developing underground cooling labyrinths as a way of storing energy. Here he writes of recent projects he has worked on that use thermal mass energy storage systems.
The recently completed Davis Alpine House at Kew Gardens is a tiny technological marvel. It is a small and seemingly simple building but it embodies some pioneering technology in the fields of glass engineering and environmental design. Developing buildings that are efficient and environmentally friendly is a key part of Kew Gardens’ brief. The design of the Davis Alpine House responds to this, eschewing the refrigerated shelves that are normally used to display and support the plants in an Alpine House.
Invisible beneath the floor of the building is a double floor that provides the stiffness to cope with forces from the tension rods that support the tall glass roof. Between the layers of floor are a series of labyrinthine concrete passageways. Air drawn from outdoors is forced to passively condition these passageways before it is fed into the glasshouse above. This passive strategy effectively uses the passageways as a heat store, to moderate swings in temperature and protect the plants from extremes of temperature.
The Davis Alpine House is the latest in a series that we have developed at Atelier Ten environmental engineers. It is innovative in the modern context but the technique draws upon some basic physical principals that can be seen in buildings pre-dating air conditioning.
Historical precedents and biomimicry
There is a group of Renaissance villas in Costozza, Italy that are connected by subterranean shafts to a series of caves that run beneath the mountains. The caves remain cool throughout the summer months by virtue of their thermal couple to the earth and their lack of exposure to sunlight. Winds blowing against the hillside drive air through the tunnel system. The air is cooled in the tunnels and then delivered to the houses through marble floor grilles, keeping the occupants comfortable on hot summer days.
Similar examples can be found in nature. The Barossa termite has perfected the building of massive structures with fully integrated passive temperature control. The system can control the temperature in the Queen’s chamber, at the heart of the nest, to within 1ºC throughout the year. The main mechanisms for this precise temperature control are based on thermal mass and evaporation. Outdoor air is drawn through tunnels into a subterranean chamber. The chamber has a large contact surface area with the ground which cools on a hot day and warms on a cold one. In extreme heat the arrangement is supplemented by evaporative cooling. The termites travel many tens of metres down tunnels to the water table to collect minute quantities of water to place into the system.
Reducing peak demands
These examples may seem a far cry from the needs of a contemporary building but the basic physical principals are transferable through many scales, and can be effective in reducing both energy consumption and peak demands on infrastructure. The potential for heat exchange with the ground through large concrete heat stores (which we call ‘labyrinths’) and through earth ducts has now been proven on a number of recent projects. However, these technologies are still rare in the UK.
The technique of exposing internal concrete surfaces within buildings in order to control internal temperature fluctuations has become commonplace over the last decade. This is ‘room coupled thermal mass’, sometimes known as the ‘thermal flywheel’ effect. By ventilating the buildings through the night the mass can be cooled down and the ‘coolth’ held in storage to absorb heat gains the following day. There is a limit to the amount of cooling that can be stored in this way and buildings using this technique can be prone to overheating. This is because the spaces containing the heat storage cannot be cooled below comfort temperatures overnight or they will require heating in the morning, thus limiting their capacity.
Labyrinths can be classed as ‘de-coupled’ thermal storage systems because the thermal mass is not coupled to the space that it is serving; it may be quite remote. The clear benefit is the ability to store more ‘coolth’ from preceding cool days and nights, thus increasing the capacity of each unit of constructed mass.
Naturally ventilated buildings get hot
In a typical mechanically ventilated building the energy required to heat or cool outdoor air, before bringing it into contact with the occupants, represents a significant energy load. In a naturally ventilated building on a hot day, the incoming hot air from outside will inevitably exacerbate overheating problems. Occupants instinctively tend to encourage breeze from windows but this will frequently make a space warmer. This is why most naturally ventilated low-energy buildings suffer from overheating. As the effects of global warming start to bite how long will it be before they are retrofitted with air conditioners?
Our first labyrinth
The first labyrinth that we completed was at the Planet Earth Gallery for the Earth Centre in Doncaster. The design allowed us to completely eliminate the need for mechanical cooling for the 6,500m2 exhibition space. The labyrinth was formed as an integral part of the raft foundations in the structure beneath the gallery floor. A series of concrete diaphragm walls were built which acted compositely as a structure, with slabs above and below. The passageways formed were 1.5m high and were also used to distribute electrical and exhibition services.
The performance of the labyrinth was simulated during the design development phase using a dynamic thermal analysis system (TAS) modelling program. An optimisation process was followed to determine the optimum area of material, the effects of wall geometry, material density and surface roughness. A number of material options were considered, from concrete to rammed earth to recycled metal. The concrete walls were used for their combination of structural and thermal performance.
Air moved through the low resistance system at very low velocity so that the energy to drive the circulation fans was minimised. A 500m2 photovoltaic array covering the main entrance generated more than enough electrical power to run the ventilation system through the year making this, effectively, a carbon neutral system. Sadly the Earth Centre closed in 2004, due to an indifferent public response.
Federation Square
Soon after the Earth Centre had been completed we went on to build a much larger labyrinth to condition air serving a huge atrium-like street at Federation Square in Melbourne. The labyrinth was formed from rippled concrete walls. These created long air paths for the air to flow down. The air is always cool at night in Melbourne and the heat of the day can be flushed out of the concrete. along with some of the moisture, so that the mass is cool the following day. The flow of air can be controlled through the various chambers to provide some responsiveness to external conditions. In addition to the thermal storage, evaporation cooling was achieved using stored rainwater on the hottest days. This method is very similar to that used by the Barossa termites.
Advanced dynamic thermal simulation modelling using TRNSYS demonstrated the temperature benefits of the techniques in place at Federation Square. It showed that it was feasible to eliminate mechanical cooling of the space, saving significant plant space and cost whilst maintaining the Atrium space at comfortable temperatures, even when 40°C outside. Materials and properties were again analysed and the rippled concrete surface was developed to increase surface contact area and roughness for maximum heat exchange. The concrete has a density of 1800kg/m3 and a specific heat capacity of 0.9KJ/kg K. Again we considered water as an alternative storage medium because of its higher thermal capacity per unit weight (SHC = 4.19KJ/kg K), but issues of how to adequately encapsulate it, and the fact that it would have no ability to act as a moisture buffer, led us to stick with concrete.
The annual energy consumption for the fans is 10% of that which would have been required for a more conventional overhead cooling system. After four full summers of operation, the scheme at Federation Square has been a great success. When cooling is not needed in the Atrium, the air is diverted to the adjacent galleries of the museum to reduce their energy demand. In the winter the labyrinth also provides some heat energy storage to reduce heat energy input.
The Alpine House
The Davis Alpine House at Kew Gardens is the most recent evolution of the idea. Wilkinson Eye Architects designed the building in collaboration with Dewhurst MacFarlane (structural engineers) and Atelier Ten (environmental engineers). Alpine plants like a cool breeze and cool roots to maintain compact foliage. They also need to be protected from intense sunlight, from too much moisture and must have as much daylight as possible. The Alpine House’s glass umbrella structure is made of single glazed sheets of low iron glass to maximise the amount of daylight reaching the plants, and to protect them from rainfall with as little obstruction to light as possible.
Two large fan-shaped retractable shades were developed especially for the building. They protect the plants from the most intense solar radiation as well as significantly contributing to visitor comfort in the viewing area. In more traditional Alpine Houses the outside of the glass tends to be whitewashed in the summer.
Flexible system
The glass sides of the building oversail the concrete base and allow air to enter at the base of the glass wall, through screened openings that keep insects and vermin out. Vents in the top chord of the structure open sequentially with increasing temperature. A natural air flow is established between the glass and the shade to liberate excess heat. In cooler weather the top vents are closed and the updraft is stopped to retain passive heat gain within the building.
The labyrinth of dense concrete block walls within the structural floor is similar to our previous constructions. The system is arranged so that a small fan can drive the air through the labyrinth tunnels and expel it outside at night, so that the structure is cool in the morning. As the building starts to heat up the outdoor air is directed straight into the glasshouse, via vertical pipes that terminate with directional outlets within the plant beds. As the outdoor temperature continues to rise above 18ºC the air is diverted through the labyrinth where it is cooled before passing through the same pipes to cool the plants. This is cooling without refrigeration. The economic appraisal at the time showed a payback period of nine years with energy inflation at 3% pa. In fact, energy prices have doubled since then and so the break-even point has been brought forward.
Thermal mass and energy legislation
Recent London (GLA), UK wide and European energy legislation requires that a proportion of the energy used in buildings be provided from renewable resources. Earth cooling and labyrinth cooling can be demonstrated to be a ‘renewable’ resource in this context.
As developers and designers wrestle with the 10% renewable energy component required by the recent GLA guidelines, the use of thermal mass systems becomes an increasingly attractive proposition. It is a less expensive and architecturally limiting way of reaching energy targets than many other alternatives.
The bigger picture
Peak loads are increasingly an issue in hot weather both in the context of our creaking infrastructure and in the debate about how to meet our future generation capacity requirements. Even if the thermal mass systems cannot completely replace comfortconditioning installations they can significantly reduce peak requirements. As temperatures increase with global warming, and the infrastructure issues associated with meeting peak electrical demands become more of a problem, the benefit of using these techniques to spread demand throughout the day and reduce peak loads becomes more obvious. Could this summer's rolling power cuts in London and the US – partly due to the energy demands of air conditioning – have been averted if more buildings had used thermal mass to delay peak loads?
The potential for tying these strategies to the more intermittent supply profiles of renewable energy systems such as wind power also represent an opportunity that is worth further analysis. For many years the potential of the earth as an energy resource for buildings has been largely ignored. It is time for us to wake up to what it offers as a way of reducing our reliance on air-conditioning and non-renewable energy.
Biography – Patrick Bellew FREng HonFRIBA CEng FEI FCIBSE MASHRAE FRSA
Patrick Bellew is a founding Director of building environmental engineering consultants Atelier Ten. He teaches environmental design at Yale University School of Architecture. Patrick has been active in the development of high performance buildings and specialises in applied thermal storage systems. He was elected a Fellow of The Royal Academy of Engineering in 2004.
Keep up-to-date with Ingenia for free
SubscribeOther content from Ingenia
Quick read
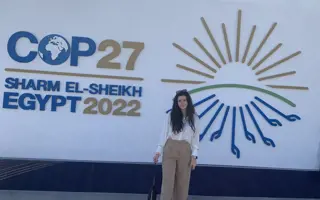
- Environment & sustainability
- Opinion
A young engineer’s perspective on the good, the bad and the ugly of COP27
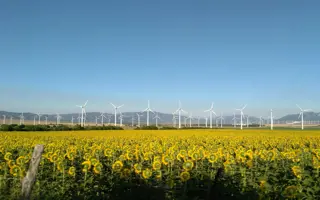
- Environment & sustainability
- Issue 95
How do we pay for net zero technologies?
Quick read
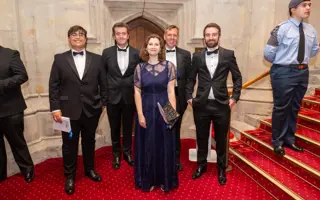
- Transport
- Mechanical
- How I got here
Electrifying trains and STEMAZING outreach
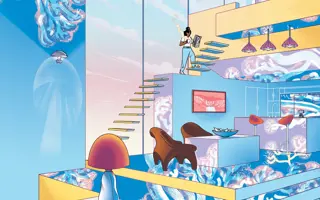
- Civil & structural
- Environment & sustainability
- Issue 95