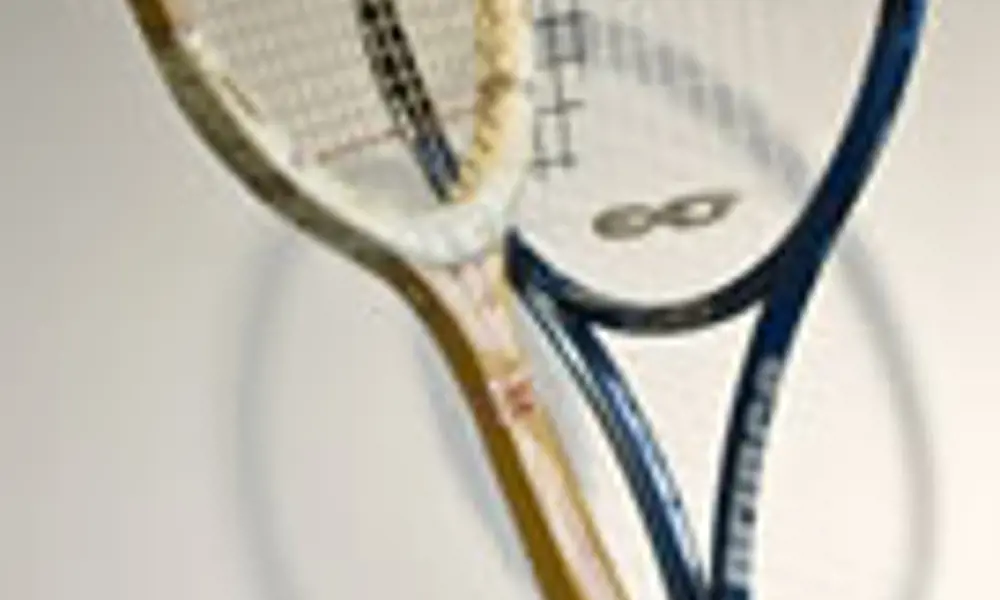
Fair Game
A 1970s wooden racket next to an example of the latest carbon fibre design which has a 40% larger head
Sports engineering has grown rapidly in scope and profile since the Second World War. Engineers havehelped improve the performance of teams and individuals so much that tension has sometimes been generated between the innovators and the regulators. Dr David James, The Royal Academy of Engineering’s first Public Engagement Fellow, is a senior lecturer in sports engineering at Sheffield Hallam University. He takes a look at the technology behind recent innovations and the knock-on effects for sport.
The term ‘sports engineering’ has only just started to enter the mainstream, but for as long as scientists and engineers have been working to understand and shape our world, the topic of sport has made for a welcome diversion. In 1672, Issac Newton commented on the spin that causes the irregular flight of a tennis ball, and over the following centuries, Heinrich Magnus and Lord Rayleigh both developed theories capable of explaining ball’s spinning properties.
The industrial revolution brought with it a transport infrastructure and new found leisure time that allowed sport, for the first time, to be pursued by a significant number of people. Early manufacturers would generally combine their main industry such as making prams, with the production of sports equipment. However, it quickly became apparent that improved performance would lead to greater sales and thus more specialist companies began to experiment with different materials and novel designs, much as they still do today.
From its modest beginnings, the sports equipment market has become a multibillion pound industry. Enhanced performance, real or perceived, lies at the heart of this industry and engineers work to differentiate their products from their competitors. The world of elite sport is almost solely driven by performance. As the staging of the London 2012 Olympics draws closer, the number of engineers striving to enhance the athletic achievements of aspiring champions has never been greater.
Skis and rackets
A key pioneer in 20th century sports engineering was the American aerospace engineer Howard Head. It has been said that Head turned ‘blaming your tools’ into an art, and with good justification. In the 1940s, Head tried skiing and to his great frustration found mastering the technique an insurmountable challenge. He blamed the very long and heavy wooden skis and went about redesigning the equipment by using his experience of laminate materials in aircraft wing construction.
Head constructed a new ski that consisted of a lightweight honeycombed core sandwiched by two thin aluminium sheets. This new design was significantly lighter than previous wooden skis and, due to the enhanced material characteristics, could be made shorter while maintaining a bending radius to allow turns. The ‘Head’ ski revolutionised the skiing industry, making it more accessible and popular. It also gave birth to a major sporting brand that still holds his name today.
Three decades later in 1975, Head found himself in a similar situation while playing tennis. Frustrated with his performance on the court, Head set about redesigning the classic wooden tennis racket. Using the latest developments in aluminium extrusion, Head developed a larger racket with no commensurate penalty in weight or reduced stiffness. This hugely popular oversize design was patented by the company, Prince Sports, and fundamentally changed the nature of the game. By the 1980s, graphite replaced aluminium as the material of choice and wooden rackets quickly became items of historical interest.
The oversize design had many significant advantages including the ability to produce more spin and increased stability for off-centre shots. Remarkably, a modern racket is around 300% stiffer and 30% lighter than older wooden rackets while having a 40% larger head. Ball speed has increased as a direct result of these innovations, so much so that ten years ago, the International Tennis Federation briefly considered the introduction of a larger ball to reduce the speed of the game.
Despite these advances, relatively little is known about how tweaking the material properties or geometry of a racket affects its performance. Manufacturers conduct their research and development by making batches of prototypes and asking professional players to provide feedback. Sometimes a ball will be fired at a test model and the impact will be observed, but in the main, the development process is very much a question of trial and error.
The leading racket-maker Prince Sports has joined forces with our Centre for Sports Engineering Research at Sheffield Hallam University to devise tools to design and fine-tune a racket to best suit individual player’s style and technique. We created a complex finite element (FE) model capable of analysing the five millisecond impact during which the ball is in contact with the racket. The model took into account real racket geometry attained from a non-contact 3D laser scanner, material characteristics attained from materials testing, and a full simulation of the 35 independent tensioned strings.
The model is able to predict all aspects of the impact including the vibrations in the racket, the compression of the ball, the deformation of the string bed and, of course, the rebound dynamics of the ball. The model has been validated extensively against high speed video data attained from both lab-based experiments and match play. The FE model is now being used as a design tool to optimise racket geometry and material properties prior to production. Despite its usefulness, manufacturers will not turn away from using their expert player feedback just yet.

Finite element model of an impact between a tennis ball and racket. This model can be used to investigate the effect of factors such as ball-to-string friction, racket mass and racket structural rigidity © Sheffield Hallam University
Engineering a performance limit
Across many sports, the athletic performance of each successive generation supersedes those of the past. Scientists and engineers both claim responsibility for this improvement but the tide of ‘progress’ is not universally welcomed and many people now believe that technology plays too great a role in any given athlete’s performance. Numerous sports have brought in new rules to curtail the potential impact of engineering on athletic performance, and many others are considering doing so. The dramatic changes to the javelin in
the 1980s are a case in point.
The origins of the javelin are clearly associated with hunting spears; however, during the 20th century the javelin was developed into a highly refined piece of equipment capable of generating large aerodynamic forces and thus attaining staggering thrown distances. The first major innovation came in 1953 with the introduction of hollow metal javelins. The increased surface area of the hollow javelin (their mass has remained constant at 800grams) allowed athletes to exploit the beneficial effects of aerodynamic lift (see box Javelin aerodynamics).
Because of the shape of these new javelins, the aerodynamic forces tend to create a pitching moment that rotated the javelin backwards in the air, opening up the javelin’s angle of attack and thus generating large lift forces. In 1984, the East German athlete Uwe Hohn exploited the javelin’s aerodynamic potential perfectly and threw an incredible 104.8 metres, beating Tom Petranoff’s previous world record of 99.7 metres. Throwing a javelin such a distance inside a stadium had clear health risks both for other competitors and spectators! Furthermore, owing to the javelin’s backwards rotation a great many throws would fail to land tip first and would therefore be disqualified. The combination of the dangerously long throws and the large proportion of ‘foul’ throws prompted the authorities to change the rules. Rather than increasing the mass of the javelin, the position of its centre of mass (its balance point) was moved forward by 4 cm. The effect was immediate and dramatic; average performances dropped by 13%.
The new javelins performed worse than their predecessors because the aerodynamic forces now acted behind the javelin’s centre of mass, thereby creating a pitching moment that rotated the javelin forwards and closed down its angle of attack. The new javelins were simply not able to generate as much lift, thus reducing the flight time. An additional benefit was that the forward rotation made tip first landings far more frequent, thereby reducing the number of foul throws.

In 1953 the javelin changed from being a wood spear into a hollowed out metal tube, from this moment the javelin became subject to significant aerodynamic forces and started to glide through the air. The enhanced design led to improvements in performances so profound that in 1984 a new rule was introduced to limit flight distance. The javelin’s centre of mass was moved in front its centre of pressure, thereby reducing aerodynamic lift. Note: Weight always acts vertically down; Drag always acts in a opposite direction to javelin velocity; Lift always acts perpendicular to javelin velocity
From 1985 to 1991, javelin performances again rose rapidly, but a second rule change to limit the javelin’s surface roughness (an important factor in reducing skin drag) effectively stopped this tide of progress.
Great swimsuit debate
In the world of swimming, a similar story has been acted out under intense media interest. Since the turn of the millennium, full body swimsuits have been worn by a seemingly endless succession of record breaking athletes. The full body suits themselves have been through a series of different designs, some of which have been more successful than others. The story started with the Speedo Fastskin – the original ‘sharkskin’ swimsuit introduced in 2000. The theory was that a fabric could be woven to mimic the effect of the miniature dermal denticles found on sharks’ skin.
Speedo superseded this design in 2007 when they introduced very smooth hydrophobic panels made of polyurethane. The impact was nothing short of remarkable, with 108 swimming world records broken in 2008. During that year, 11 swimmers completed the 100 metres in less than 48seconds, whereas only one swimmer had managed the feat between 2000 and 2007.
This tidal wave of records continued in 2009 with the introduction of full polyurethane suits and fierce competition between rival manufacturers. The swimsuit saga reached its climax during the World Championships in Rome when an incredible 43 world records were broken during the eight day competition and public support for the technology all but evaporated. Later in the same year, FINA (the world governing body for swimming) banned the use of non-textile full body swimsuits and the term ‘technology doping’ firmly entered the sporting vocabulary.
There is surprisingly little independent peer reviewed research that has been able to explain what was so special about the swimsuits released between 2007 and 2009. These suits all incorporated the hydrophobic material polyurethane and thereby reduced the magnitude of skin drag. The suits were also worn incredibly tight, and there is evidence to suggest that squeezing your body into a very tight garment has a number of potentially beneficial physiological effects. And finally, it has been argued that the tight suits were able to trap air between the swimmer’s skin and the non-permeable fabric, thereby increasing buoyancy.
It is likely that to a greater or lesser extent, all these effects contributed to the step change in performance seen in 2008 and 2009. The new rule change implemented by swimming’s governing body FINA in 2010 effectively removes the possibility of using, refining or developing any of these interesting swimsuit advances. From a sports engineering perspective, this is very disappointing, but it is also typical of the reoccurring conflict between the innovator and the regulator, a conflict that shows no signs of abating.
The implications of this technological roll back are difficult and manifold. Will swimmers ever be able to exceed the records set during the era of technological enhancement? Should the world records set during this period be nullified? Does swimming run the risk of stagnation with little chance of progress?
Fastest man with no legs
One of the most emotive and controversial technological issues facing the world of sport today concerns the use of running prosthetics by amputees. Since its inception at Stoke Mandeville Spinal Injuries Centre after the Second World War, the Paralympics have been intimately linked to science and engineering. New technologies have enabled individuals with profound disabilities to compete at the very highest levels, thereby challenging society’s prejudices and promoting inclusion. Sports engineering has usually been warmly welcomed into this field, but recently, it has come under attack again.
For most elite amputee runners, the job of their running prosthetic is to match the gait of their remaining leg. In this case there is little opportunity for the engineer to significantly enhance the performance of the prosthetic as it would fundamentally unbalance the runner. However, there are a small number of elite bilateral amputees where such constraints are not applicable. One such athlete is Oscar Pistorius, the world’s fastest man with no legs, and one of the greatest ever Paralympians. Pistorius has stirred up a hornet’s nest by wishing to compete against non-disabled runners in IAAF (world governing body for athletics) sanctioned events. Pistorius specialises in the 400metres, an event in which he uses the ‘Cheetah’ running blade developed by the orthopaedic company Ossur.
A number of studies have looked at the running efficiency of Pistorius both from biomechanical and physiological perspectives. Based on these studies, the IAAF banned Pistorius from competing against non-disabled athletes as he was deemed to have a significantly lower (almost 30%) energy cost while running. The blades are made of carbon fibre and have a lower mass than muscle and bone; they are also elastic and allow the runner to ‘bounce’ with little energy cost. A non-disabled runner does not ‘bounce’ without having to actively push off the ground, thereby incurring an energy cost.
After the initial ruling by the IAAF, there was considerable debate and disagreement about aspects of the fundamental science, but perhaps more importantly, public opinion was overwhelmingly against the IAAF and in support of Pistorius. In 2008, just a few months before the start of the Beijing Olympic Games, the case was taken to the Court of Arbitration for Sport in Lausanne where the IAAF ruling was revoked. The only barrier left was for Pistorius to make the Olympic qualifying time, a feat that proved to be just too great for this exceptional individual. However, fast forward a couple of years and Pistorius is on track to qualify for the London 2012 Olympics. He recently ran a personal best of 45.61, just six hundredths of a second slower than the qualifying time.
Today, the scientific community have largely overcome their differences and reached a consensus view that running blades provide a significant advantage to a bilateral amputee, particularly over the longer running distances where the acceleration phase is not so important. Nonetheless, the door to non-disabled competition remains open to any disabled athlete who is capable of achieving the qualifying time. One has to consider what the public reaction would be if a disabled athlete were to actually win a race against non-disabled athletes? It is highly unlikely that public support would remain as strong for the enabling prosthetic technology and a total ban or enforced performance limitation would undoubtedly be introduced (see Box: Oscar Pistorious)
Faster, Higher, Stronger
Throughout the history of modern sport, a battle has been waged between the engineer and the regulator. Enhancing performance by using technology can challenge the traditions and culture of a sporting practice. While some sports welcome the opportunity to change and evolve, others are rooted to their traditions and can aggressively knock back engineering innovations.
Ultimately it is the viewing public who define the culture of a sport, and the decisions made by governing bodies generally reflect this adopted culture and its associated values. However, each sport has its own distinct identity and should be allowed to pursue its own future in whichever way it feels is best. While there are specific regulations on the use of performance-enhancing drugs as enforced by the World Anti-Doping Agency, there are no universal values or rules with regard to the use of engineering in sport. It is my personal opinion that this should remain the status quo.
Work is now being undertaken by Sheffield Hallam University to complete an in-depth performance analysis across all sports where reliable historical performance data is available. The aim is to understand what changes in performance have been achieved through engineering, and what has been achieved through pure athletic endeavour. It is hoped that this knowledge will aid the decision-making of those charged with setting the rules of sport, and lead to fewer reflex reactions in terms of what technologies are permissible or not.
Sports engineering is often attacked on the grounds that it is making sport ‘easier’. While it is evident that new technologies do help athletes achieve the Olympic motto ‘Citius Altius Fortius’ (Faster, Higher, Stronger), to suggest that modern sport is ‘easier’ is to fundamentally misunderstand the nature of competition. It is just as hard to win Wimbledon today as it ever was, but at the same time, quite impossible to win with a wooden racket. Traditionalists may disagree, but sport is arguably best served if it can evolve and progress alongside our rapidly changing world. Moving too fast can cause major difficulties as was the case with the javelin and full body swim suits, but equally, standing still can lead to stagnation. The trick, of course, is to run at just the right speed.
Keep up-to-date with Ingenia for free
SubscribeOther content from Ingenia
Quick read
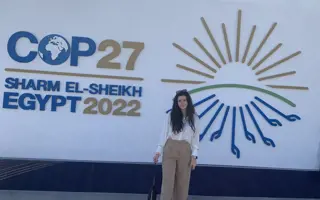
- Environment & sustainability
- Opinion
A young engineer’s perspective on the good, the bad and the ugly of COP27
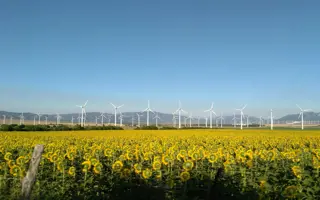
- Environment & sustainability
- Issue 95
How do we pay for net zero technologies?
Quick read
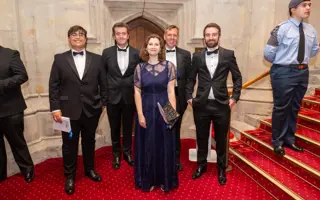
- Transport
- Mechanical
- How I got here
Electrifying trains and STEMAZING outreach
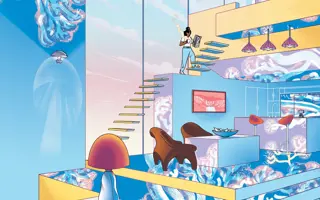
- Civil & structural
- Environment & sustainability
- Issue 95