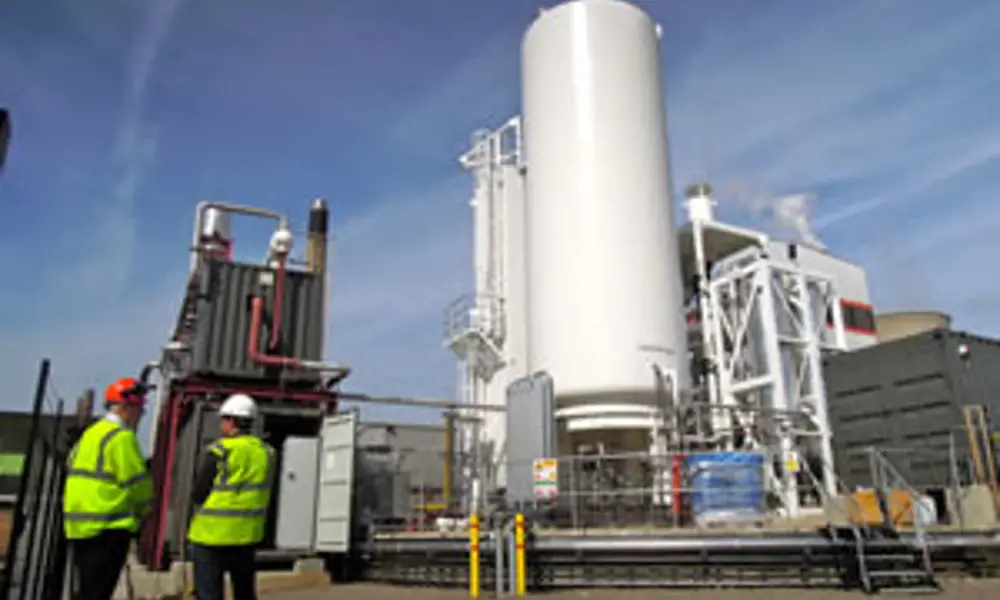
Energy storage technologies
The Highview cryogenic energy storage demonstrator plant in Slough
Energy storage already plays an important role in balancing supply and demand across the electricity grid. With an increase in intermittent generation, the flexibility provided by using new forms of stored energy has the potential to both reduce the costs and improve the security of the overall system. Dr Jonathan Radcliffe looks at some of the technologies being considered that could enable future energy storage systems
Unlike most manufactured goods and commodities, energy, especially electricity, is not easily stored after it has been generated. The ‘stores’ of primary energy have traditionally been reserves of oil, gas, coal and fissile material, with most energy systems holding a relatively small amount of hydroelectric power behind dams. The result is that power suppliers have usually installed generating capacity to meet peak demand, with a safety margin for breakdowns and maintenance. One consequence of having significant amounts of intermittent renewable energy is that power stations would run at capacities lower than they currently operate at.
Governments and public bodies are now actively considering energy storage as a way of providing greater flexibility for systems that will have new forms of generation and changing patterns of demand.
Storage Strategies
In the UK, the government forecasts that, by 2020, the country will have installed wind turbines that can generate 31 GW of electricity (18 GW offshore, 13 GW onshore). Recent signs suggest that ministers are also keen to accelerate the take-up of photovoltaic solar cells and to have 7 to 20 GW of capacity installed by 2020. This means that, with a total expected installed generating capacity of 110 GW, a significant proportion of our electricity will come from intermittent sources.
The Department of Energy and Climate Change (DECC) explained in its 2012 report UK Renewable Energy Roadmap Update how greater use of energy storage could help in the takeup of renewable energy, such as wind power and solar photovoltaic (PV) power, saying “Innovation in cost-effective storage solutions and grid management could help bring about a step-change in affordability and long-term potential for solar PV in the UK in this context.”
The electricity network must match – or ‘balance’ – supply and demand on a real-time basis. Balancing a network with more intermittent generation demands the greater flexibility in other parts of the system that energy storage can bring. A number of technology options and opportunities could provide this flexibility. These energy storage technologies can be characterised by the power (inkW or MW) output over a period of time (normally hours,h) to give the total amount of energy (in kWh or MWh) that the store can deliver.
Energy is normally converted from one form to another so that it can be stored, then converted again after storage. For example, electricity can produce hydrogen and store energy chemically, or it can pump water uphill into a reservoir, storing ‘potential’ energy. Reversing the process can then generate electricity. These conversion processes are not perfect: a system will always give back less energy than was used to store it, with the ratio being measured as its ‘efficiency’. The percentage efficiency can be important for reconciling the value of storage against its costs.
We can categorise the technologies used to store energy, and the conversion processes involved, broadly as mechanical, electrochemical, chemical, thermal and electromagnetic. Each discipline has its advocates, with researchers pursuing different approaches. Considering the power, energy, efficiency and physical characteristics of a technology helps us to understand how it could play a role in the energy system.
Mechanical
The most obvious ‘mechanical’ technology is the pumped storage used in hydroelectric schemes. Pumped hydro accounts for 99% of current energy storage with around 130 GW worldwide. This approach uses ‘off-peak’ electricity to pump water from one reservoir to a higher one where it has greater potential energy. The dam releases water to spin turbines and generate electricity as needed. Such schemes recover around 70% of the energy put in. A typical pumped-hydro installation has a power output from 250 MW to 1.5 GW, discharging its stored energy over a period of up to 10 hours, with the ability to store up to 14 GWh. The main constraint to this method is the mountainous terrain needed to make it work.
Compressed-air energy storage (CAES), created by pumping air into a reservoir, conventionally uses underground caverns, though vessels above ground can also store energy. To recover the stored energy, compressed air is discharged into a gas-fired combustion turbine. Plants in Germany and the US have already achieved outputs of up to 400 MW over 10 hours.
Alternative approaches to CAES also store energy from the heat produced when a gas is compressed. The heat is held in a thermal store and reintroduced into the gas when it is expanded to drive the turbine, thus requiring little or no fossil fuel. Both methods, storing compressed air and heat, can give efficiencies of over 70%, but the need for geologically suitable underground reservoirs limits this technology.
Another mechanical approach to capturing off-peak energy is to spin flywheels that can store kinetic energy. Flywheels can be very responsive, delivering large amounts of power over short timescales to provide frequency regulation. New materials and magnetic levitation for bearings allow flywheels to store more energy by spinning faster, up to around 20,000 rpm, and have lower losses, with 93% efficiency in energy recovery and 20-year lifetimes. Combining individual units can provide up 20 MW over 30 minutes.
Electrochemical
Electrochemical energy storage, in the shape of the ubiquitous battery, is perhaps the most familiar way of holding energy in reserve. Batteries are rapidly becoming part of the armoury of large-scale energy storage, with a number of competing technologies.
Redox flow batteries work by reversible reduction-oxidation, using electrolytes stored as liquids in two separate tanks. The vanadium redox battery (VRB) is the most advanced type, with vanadium dissolved in dilute sulphuric acid and held with different valence in tanks. Zinc-bromine batteries have also been commercialised. Scaling up energy storage is a case of changing the size of the tank. Individual units can be scaled up to 6 MWh, delivered over two to eight hours, with long lifetimes and efficiency around 70%.
One of the most widely used commercial storage technologies is the sodium-sulphur battery, with 300 MW deployed globally. Sodium-sulphur batteries are high-temperature electrochemical devices with molten sodium and molten sulphur as electrodes. These can achieve 80% efficiency, with 15-year lifetimes.
Lithium-ion (Li-ion) batteries are a standard technology for portable consumer devices and some electric vehicles. They are made up of electrochemical cells, usually with lithium cobalt oxide as a positive electrode and carbon for the negative electrode. Li-ion batteries are light, with a number of features that can alter power/energy characteristics. They can have 90% efficiency and a good charging cycle life. Some electricity distribution networks are testing Li-ion batteries, with Scottish & Southern Energy (SSE) testing a 25 kW device that can store 25 kWh, and UK Power Networks (UKPN) one at 200 kW/200 kWh.
Lead-acid batteries, perhaps the oldest commercial battery technology, continue to be developed with new materials. As a mature technology with relative low cost, lead-acid batteries are an option where space is less limited. They are being tested on grid applications up to 20 MW.

BYD and the State Grid Corporation of China have built the largest grid battery storage facility in the world. Located in Zhangbei, Hebei Province, China, the LiFePO4 battery combines 140 MW of renewable energy generation (both wind and solar), 36 MWh of energy storage and a smart power transmission system
Chemical
Chemical storage, apart from batteries, has also been brought into the quest for ways of stashing off-peak power for later use. Hydrogen production from electrolysis of water using off-peak or surplus power, or from gasification of coal as part of a carbon-capture plant, allows energy to be stored, transported and used through multiple pathways. Though the process may be relatively inefficient, as a proven technology that can decarbonise difficult sectors, it could be valuable.
One advantage of a ‘hydrogen economy’ is that it could deliver a portable fuel to replace hydrocarbons in transport. Another option is to feed hydrogen created from renewable electricity sources into the gas grid to decarbonise heating. The gas grid is itself a large and effective store, three times the size of the power grid in energy terms.
Electromagnetic
There are also ways of storing electricity itself, in superconducting systems, for example, or in capacitors. Their characteristics make them valuable for maintaining electricity supplies within transmission standards. Superconducting magnetic energy storage (SMES) systems circulate a current round a superconducting circuit. For the material to remain a superconductor, it has to be kept at very low temperatures with liquefied gases, usually helium, at a temperature of -269 °C, or, in the case of so-called high-temperature superconductors, with liquid nitrogen at -196 °C. Superconducting storage systems can deliver a high power output, within a second, for short periods.

A superconducting magnetic energy system (SMES) stores energy in the magnetic field of a superconducting coil, which needs to be kept at low temperature
Capacitors store energy in the electric field between two charged plates. Electrolytic double layer capacitors (EDLC), or supercapacitors, have porous electrodes with a liquid electrolyte, blurring the distinction between conventional capacitors and electrochemical batteries. EDLCs have high power but low energy densities, with low response times and good cycling characteristics. They can be used in conjunction with batteries as part of hybrid storage systems for electric vehicles.
Thermal
Just as heat is an intermediary in most conventional approaches to electricity generation, it can also play a role in energy storage. For example, liquefied air can hold energy in reserve for times when demand is high. The principles behind using liquid air for energy storage are straightforward: electricity powers an air liquefaction plant with the resulting liquid, predominantly nitrogen at -196 °C, stored in an insulated container. When power is required, the liquid is re-gasified at high pressure to drive a turbine and generate electricity.
Interest in this technology has been growing. The Royal Academy of Engineering and the Centre for Low Carbon Futures are organising a conference to discuss whether liquid air could provide a credible alternative to existing fuel systems.
At the opposite end of the spectrum, energy is stored as heat for a range of applications. Concentrated solar power can focus sunlight using parabolic mirrors to heat molten salt during the day. The molten salt is then stored in a insulated container, from which its heat can be extracted at night to boil water and drive a steam turbine. Electricity can also be generated from heat stores using heat pumps and heat engines – see Thermal process.
Given that heating buildings accounts for 42% of non-transport energy demand in the UK, storing heat and using it directly to provide thermal comfort in our homes is one serious option being widely considered. Heat can be stored by raising the temperature of water, but a hot water cylinder holds just a fraction of the heat a house would need to stay warm. Alternative thermal storage technologies are in testing which have a higher energy density than water. For example, ‘phase change’ materials, such as paraffin, can store or release heat as they change from solid to liquid, holding several times the amount of energy compared to the same volume of water.
Recent Developments
There is now a large range of storage technologies available or in development. As well as further improving the performance levels of each approach, there needs to be a broader, whole energy systems, view. With this in mind, it helps to think about the service that the energy provides. For example, for buildings we would need thermal comfort, so rather than storing electricity to run a heat pump, a solution might be to store heat.
The 2012 report for the Carbon Trust, Strategic Assessment of the Role and Value of Energy Storage Systems in the UK Low Carbon Energy Future, found that with a high proportion of renewables in the energy mix, storage could be more valuable than previously suggested. The report explains that this is because its analysis “includes savings in generation capacity, interconnection, transmission and distribution networks and savings in operating cost”.
In January 2013, the Rt Hon David Willetts MP, Minister of State for Universities and Science, picked out energy storage as one of eight “great technologies” for the UK, putting forward £30 million “to create dedicated R&D facilities to develop and test new grid-scale storage technologies, helping the UK capitalise on its considerable excess energy production, saving money and reducing the national carbon footprint”.
For its part, the Department of Energy and Climate Change is funding innovation around heat and electricity storage, including £17 million for a demonstration plant to address grid-scale storage and balancing needs in the UK electricity network. Research Councils UK also see energy storage as a priority for funding, with two large projects underway to investigate new technologies and how they could be used in future energy networks. However, this is a global challenge and there will be a need to engage internationally.
Energy storage could even be a factor in the international trade of energy. Opportunities for development of pumped hydro storage may be limited in the UK, but Norway could provide balancing power to its near neighbours. The largest, hydroelectric reservoir in Norway, Lake Blåsjø, stores 7.8 TWh, almost 1,000 times that of the UK’s largest pumped storage scheme at Dinorwig in Wales. Coupled with offshore wind, and the existing transnational collaboration to build a North Sea grid, which includes the UK and most of the countries around the North Sea, this option could reduce the overall system costs of deploying renewables.
Energy storage offers significant export opportunities. The potential global market for energy storage could be hundreds of billions of dollars. There is already a growing market for storage, and even if its size relative to generation capacity were merely kept at historic levels.
The UK is now in transition between an era where energy supply and demand have been predictable and easily managed, and a period in which they will need more active management. The technology options have to be there, but so does the policy and regulation framework to encourage investment. The UK is in a good position to move quickly in this field, but this will depend on policy decisions based on a long-term and whole-systems view of our energy infrastructure.
Keep up-to-date with Ingenia for free
SubscribeOther content from Ingenia
Quick read
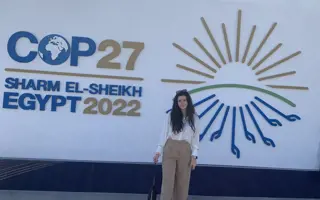
- Environment & sustainability
- Opinion
A young engineer’s perspective on the good, the bad and the ugly of COP27
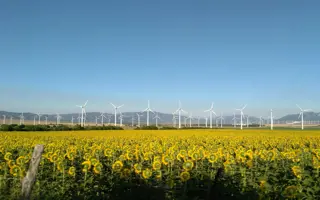
- Environment & sustainability
- Issue 95
How do we pay for net zero technologies?
Quick read
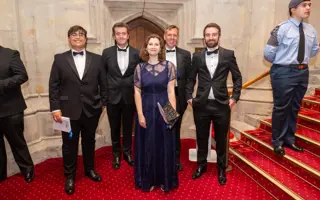
- Transport
- Mechanical
- How I got here
Electrifying trains and STEMAZING outreach
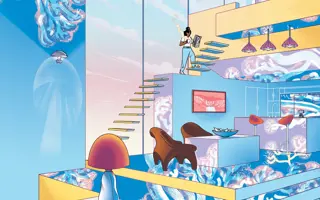
- Civil & structural
- Environment & sustainability
- Issue 95