
Design-led innovation and sustainability
Located in Athens, Greece, the Stavros Niarchos Foundation Cultural Center (SNFCC) is a modern monument to the values of culture, learning and humanity. The new cultural centre is funded entirely by the philanthropic foundation established in 1996 to perpetuate the legacy of the wealthy Greek shipping magnate Stavros Niarchos. The Stavros Niarchos Foundation (SNF) supports projects that are expected to achieve ‘a broad, lasting and positive impact for society at large’.
It was this vision that led to two important drivers for the project’s design. The SNF wanted something that would inspire and benefit all Greeks, not just the minority of opera buffs and bibliophiles. This meant extending the uses of the building, and making it and the surrounding park as accessible and attractive to the public as possible. Secondly, from the start, there was a determination to achieve the highest levels of sustainability. A target was set (which has now been achieved) to make this one of the first buildings in Greece to achieve the US Green Building Council’s LEED (leadership in energy and environmental design) Platinum standard. This was made difficult by Athens’ hot and humid summers, and by the overriding need to conserve scarce water supplies.
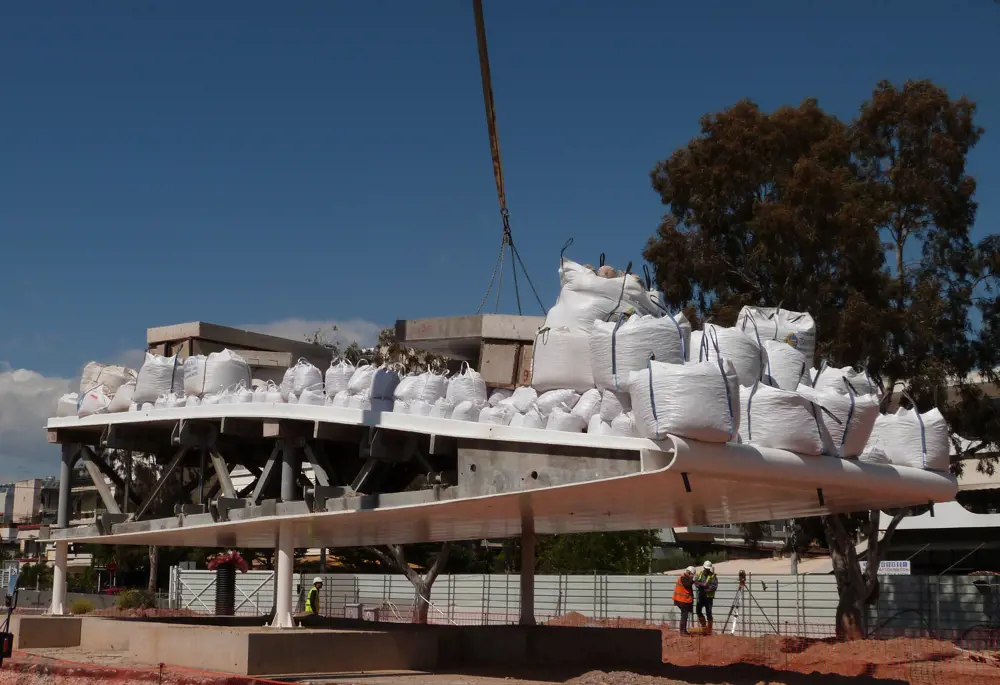
The prototype section of the canopy with its twin ferrocement shells and steel bracing being load tested in Athens. When the structure safely survived its anticipated failure loading, the team added concrete blocks to see when and how it would collapse, but they did not induce failure © Expedition Engineering
A dramatic view
The site chosen was a large plot (to include a 21-hectare public park) in the southern district of Kallithea, which translates as ‘beautiful view’. Kallithea was once a major port for Athens, but rapid urban development in the 20th century detached the site from the water. The project’s architect Renzo Piano resolved to restore this connection visually if not physically. He conceived the idea of an artificial hill on which a sloping park would be created, rising 30 metres to a large public space on the roof of the opera house, with views out across the water, and a view in the opposite direction to the ancient Parthenon, an earlier monument to Greek learning and humanity. He also envisaged a huge 400-metre-long by 30-metre-wide seawater ‘canal’ along the south-west side of the park, prevented from physically connecting to the sea only by a modern ring road. This would also help to create a cooler microclimate for the site.
A key to meeting the sustainability objectives was to make the building as close as possible to carbon neutral. This meant a combination of designing the building and its systems to use as little energy as possible, and harnessing renewables to provide a substantial part of that energy. For the latter, the engineers made a bold claim at the design team’s first workshop at the start of the project: with Athens’ intense sunlight, a sufficiently large array of photovoltaic (PV) cells could provide all the renewable energy that the building required, thus eliminating the need for unsightly wind turbines or less efficient ground source heat pumps. If the cells could be mounted on a canopy over the opera house, this could also provide shade for the rooftop public space and help reduce solar gain in the building below, as well as providing a striking distinguishing feature for the centre, visible from a distance. The canopy became a key feature of the project.
A key to meeting the sustainability objectives was to make the building as close as possible to carbon neutral. This meant a combination of designing the building and its systems to use as little energy as possible
The engineers’ initial concept was for a tensegrity structure – one where rigid struts taking compression are connected by thin cables that are always in tension, which produces a light structure with struts that appear to float in space. With help from the University of Cambridge, where a prototype was created and a PhD thesis prepared, all was proceeding satisfactorily and by summer 2010 the final construction design phase was just starting, but the architect Renzo Piano was not happy. In the 1970s, he had made his name (with Richard Rogers and Arup) designing the Centre Pompidou in Paris, with a design that turned the building inside out and introduced the public to exposed services and structure. With the SNFCC he wanted the opposite: a simple canopy, with no overt details of struts, cables or associated services.
Piano called a meeting of key members of the design team to his head office in Genoa, Italy, to brainstorm a solution. He wanted a structure that would be durable in a marine environment, support the weight of the PV panels under seismic loading, and protect against sun and rain, with a perfectly smooth underside with no visible joints and an exceptionally thin edge. It had to ‘float’ over the opera house with a minimum of heavy columns. It had to be capable of being built, and demonstrably so in order to give contractors sufficient confidence to submit competitive tenders. The design also had to be complete and ready to go to tender within eight months, as the client was not prepared to delay.
History of ferrocement
🌼 The invention of a gardener that pre-dated concrete and helped form the Rome Olympic buildings
Ferrocement was invented by French gardener Joseph-Louis Lambot in the 1840s. He found that by embedding fine meshes of iron wire in a thin layer of hydraulic cement/sand mortar, he could produce a material that was light, strong, cheap, water resistant and easy to form in irregular shapes. He recommended his ‘ferciment’ as a replacement for wood anywhere that water resistance was important, such as floors, water tanks and planters, and he used it to build a rowing boat. His invention pre-dated reinforced concrete.
However, producing the wire mesh at that time was labour intensive, and the production of larger diameter bars, and later the introduction of steel bars, led to ferrocement being overtaken by reinforced concrete and largely forgotten for a century.
The idea was picked up by Italian structural engineer Pier Luigi Nervi who was commissioned during the Second World War to design ferrocement boats. In 1945, he built one of his own – a 165-foot-long yacht with a hull 35 millimetres thick. He included ferrocement elements in his subsequent buildings, such as his celebrated 94-metre-span Hall B for the Turin Exhibition in 1948-49, and later used the material as permanent formwork for buildings including those for the 1960 Rome Olympics.
Since then, ferrocement has had a niche following among engineers who appreciate its fine qualities: potentially cheap and simple to make with no formwork required (although formwork was used in Athens), surprisingly elastic, resistant to cracking because of the close spacing of the steel wires (and hence durable when wet), and easily formed into complex shapes. Applications have included: specialist roofs, notably in the Middle East; water tanks in India; low-cost housing in the developing world; large-scale sculptures; ‘concrete canoes’ built and raced by engineering students throughout the world since the 1970s; and low-cost yachts, particularly those built by enthusiasts such as the SNFCC’s architect Renzo Piano. The canopy for the SNFCC in Athens is the world’s first ferrocement structure of this scale.
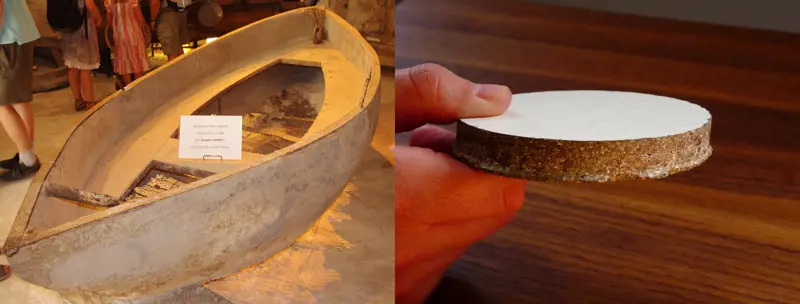
Joseph-Louis Lambot’s ferrocement rowing boat
© Jorune, Wikimedia Commons (right) Demonstrating the thinness of the SNFCC’s shell © Expedition Engineering (left)
Finding ferrocement
Options for the surface finish including plywood, steel, aluminium, ceramic and conventional reinforced concrete were debated and discarded, and eventually ferrocement (ultra-thin reinforced concrete) was chosen. It was a bold choice: apart from Renzo Piano, who had built his yacht in ferrocement, none of the design team had direct experience of the material. Although it was invented in the 1840s and used intermittently since the 1940s, it had never been used in the way envisaged for the cultural centre. Expertise and research were limited, there were no appropriate codes for guidance, and time was quickly running out.
Expertise and research were limited, there were no appropriate codes for guidance, and time was quickly running out
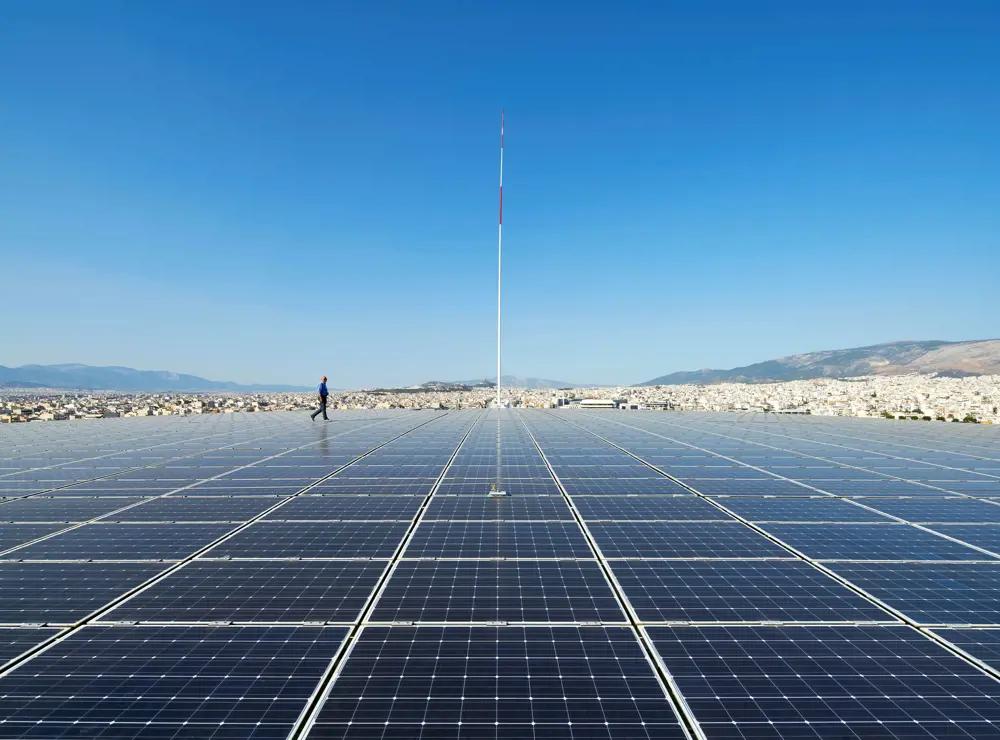
The tight packing of PV cells on top of the canopy was achieved by laminating the panels so that they can be walked on, which removes the need for maintenance walkways © Michel Denancé
Initial evaluation showed just how suitable the material was in this context. It is straightforward to construct, with multiple layers of wire mesh, a conventional cement/fine sand mortar that covers the mesh by just three millimetres, and the top surface is finished with a plasterer’s trowel. It is highly durable and requires no over-cladding panels, which could degrade, warp or deteriorate over a long design life. It is well suited to prefabrication and seamless splicing: the small diameter (as little as 0.8 millimetres) and close spacing of the wire mesh and bars means that only small overlaps are needed to maintain structural continuity over the splices. Plastering techniques help to create smoothness across joints. It can be formed into curved shapes and – most importantly – it is (at only 20–25 millimetres) an order of magnitude thinner than an equivalent reinforced or post-tensioned concrete structure. It also has a better strength-to-weight ratio, so reduces the load on the opera house beneath.
Adding to the challenges of design and analysis were the non-linear characteristics of ferrocement, including micro-cracking and long-term creep, as well as the arduous loading criteria

A particular challenge was how to run a large and inflexible high-voltage cable down from the PV cells on the canopy, invisibly and allowing for the large movements of the roof. The solution was an elegant spiral sculpture, which happens to support and contain the cable and effectively absorbs any movement © Michel Denancé
Some UK expertise was brought on board, notably independent consultant Patrick Jennings and the University of Manchester’s Paul Nedwell, and the project’s engineers learned the artisan skills of making ferrocement samples in the university laboratories. More than 100 large panels were then produced for extensive testing at the National Technical University of Athens.
Meanwhile, the structural form was developed: an upper and lower shell separated by hidden steel bracing spanning in both directions, with shells coming together at the perimeter to produce the sharp edge required by the architect. Adding to the challenges of design and analysis were the non-linear characteristics of ferrocement, including micro-cracking and long-term creep, as well as the arduous loading criteria, particularly from large horizontal and vertical seismic movement. No suitable software was available, so the engineers developed their own in-house software from first principles. To convince everyone, particularly sceptical contractors, that it was buildable, a full-scale 15 metre by 6 metre prototype of a section of the main canopy was constructed and load tested by local labour.
Springs in the sky
🌞 The loading challenges and engineered solutions for the solar canopy
The 100 metre by 100 metre solar canopy is inherently a very stiff structure that has to cope with a number of loading challenges: wind loads that can create very high local stresses, with large forces on the columns; large seismic loads, both vertical and horizontal; differential and variable settlement of the opera house underneath; and, in particular, the ‘banana’ effect as the top skin warms and expands in the sun, tending to pull the centre of the structure upwards.
To tackle these factors, the engineers came up with a novel scheme for a ‘machine’ in which a system of springs and dampers connects the head of each of the 30 columns to the canopy in a ‘soft’ manner. This effectively reduces internal stress concentrations in the canopy and reduces the peak reactions on the roof of the opera house by as much as 40%, therefore reducing the structure needed within the opera house to transfer the load to the foundations. It also optimises the dynamic performance of the canopy under wind or seismic loading, and increases the predictability of the internal forces for which the ferrocement is designed.
Conventional structural analysis software is not well set up for understanding and developing such mechanical systems, so physical modelling was central to the design process. This began with modelling in Meccano, followed by bespoke numerical kinematics modelling, 3D printing with mechanical springs, developing a full design in BIM (building information modelling), and finally building and testing the column head system at full scale.
Each column head includes a stiff steel frame fixed to the ferrocement, four springs providing for movement up to +/-150 millimetres, dampers to dissipate energy from wind and seismic movement, and low-friction bearings for robustly and rigidly carrying large lateral loads. At the suggestion of the contractor’s engineers, fluid polymer springs were chosen, adapted from the buffers of French TGV (high speed) trains. This had the added advantage that, on the completion of construction, the act of pumping the fluid polymer into the springs allowed the canopy to lift itself from the temporary scaffolding quickly and safely.
Sensors within the column heads continue to be monitored by telemetry, validating predictions, including an actual 100-millimetre bowing movement under differential thermal loads.
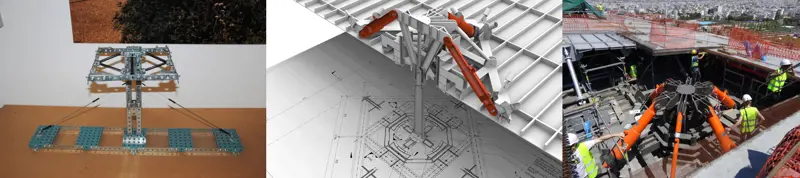
The column-head springs to support the canopy: an early Meccano model to test the concept (top); a 3D model to aid construction (middle); and a completed column head in place (bottom) before installing the ferrocement panels over the top. The large orange elements are the fluid polymer springs © Expedition Engineering
Efficient design
The ‘floating’ effect 14 metres above the opera house is created by supporting the canopy on 30 slender cylindrical steel columns, braced by diagonal cable ties. Designing a connection detail between the column heads and the canopy proved the greatest engineering challenge of all. All of the inverters and transformers for capturing energy from the PV cells and converting it to high voltage are contained and hidden within the canopy.
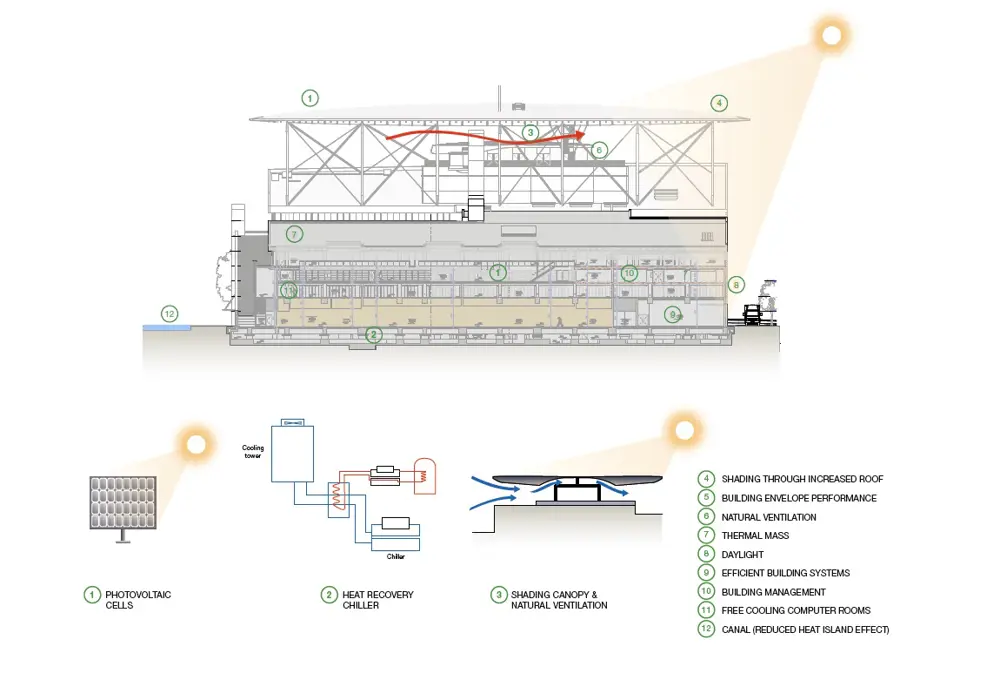
The 12 key elements of the sustainability strategy for the SNFCC building © Michel Denancé
Water self-suffficiency
💧 Irrigating the 15-hectare sloping park without drawing on scarce water supplies
Athens’ climate combines hot, dry summers with flood-risk storms, which created challenges for irrigating the 15-hectare sloping park without drawing on scarce potable water supplies. It led to the development of innovative water and drainage solutions including SUDS (sustainable urban drainage system) and rainwater harvesting.
The first measure to conserve water was the choice of plants for the park: native Greek species and other Mediterranean plants that are relatively tolerant of dry conditions and require minimal irrigation. The principal water source is brackish groundwater from four boreholes on the edge of the park, which has to pass through a desalination plant before use. This is supplemented by seawater drawn from the bay and pumped across a bridge over the ring road to the site for desalination. The seawater is also used to refresh the ‘canal’ that runs along one side of the site.
All of the rainwater falling on the canopy over the opera house is caught in an invisible gutter along the perimeter, channelled within the canopy, dropped down pipes inside the steel columns supporting the canopy, and carried to buried gravel trenches located throughout the park that infiltrate the rainwater and allow it to recharge the aquifer below the park.
Stormwater in the park, particularly from the hard surfaces of the paths, is collected in drainage channels and carried to the gravel trenches and a set of buried, geotextile attenuation tanks. These serve two purposes: they absorb much of the water from a peak storm, so prevent overload on the local stormwater drainage system; and they allow the stormwater run-off to permeate the ground and also recharge the aquifer. This is designed to cope with anything up to a 1-in-50-year storm. For anything greater and up to a 1-in-200-year storm, part of the park would be allowed to flood. Water from the seawater canal would rise to inundate the surrounding paths, but not the cultural centre itself.
Within the buildings, water demand is reduced by measures such as low-flow appliances and fixtures and waterless urinals. Grey water (all waste except from toilets) from the opera house is collected, filtered, and where necessary treated, stored and then recycled for uses such as toilet flushing.
Altogether these measures reduce the development’s impact on the local water utility infrastructure by 37,000 cubic metres, equivalent to 15 Olympic swimming pools per year.
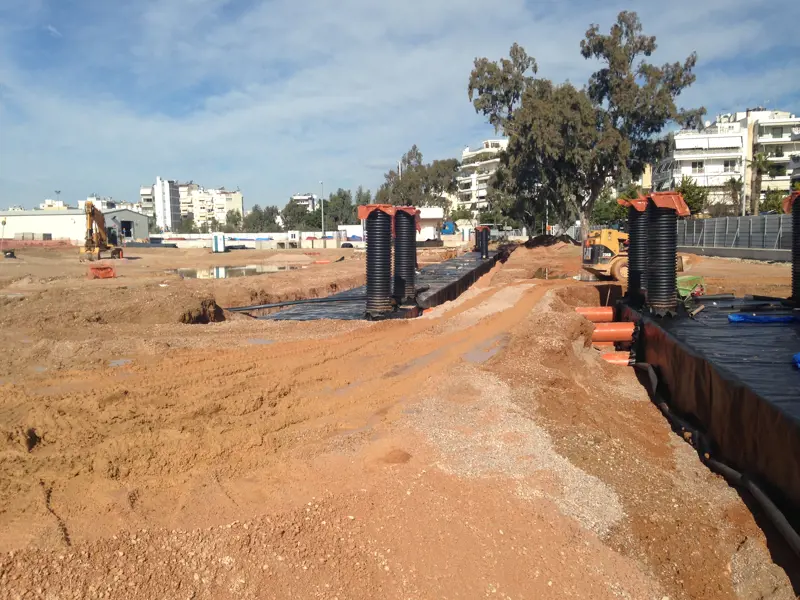
Onsite surface water attenuation is provided throughout the park in the form of geocellular tanks and deep gravel trenches. Both act to store water during times of heavy rainfall, which protects the municipal drainage system from being overloaded and allows for infiltration to recharge the aquifer © Michel Denancé
Two factors threatened to limit the power output from the PV cells on top of the canopy: the limited efficiency of available units, and the need to leave space between the cells for maintenance walkways. The first was addressed by specifying outputs beyond what could be achieved, in the (correct) expectation that designs would improve by the time of procurement. The second led to a laminated glass coating, allowing each cell to carry the weight of two booted workers carrying a spare cell. The glass slightly reduced the efficiency, but this was heavily outweighed by allowing the cells to be closely packed with just a small separation for air flow. The final result is a 100 metre by 100 metre PV array made up of 5,560 panels delivering 1.3 MW (megawatt) of power, and expected to generate 2 GWh (gigawatt hours) per year, similar to powering 650 UK family homes. Additional power will be required from the grid at peak times, but at other times the PVs will feed surplus power into the grid.
Two factors threatened to limit the power output from the PV cells on top of the canopy: the limited efficiency of available units, and the need to leave space between the cells for maintenance walkways
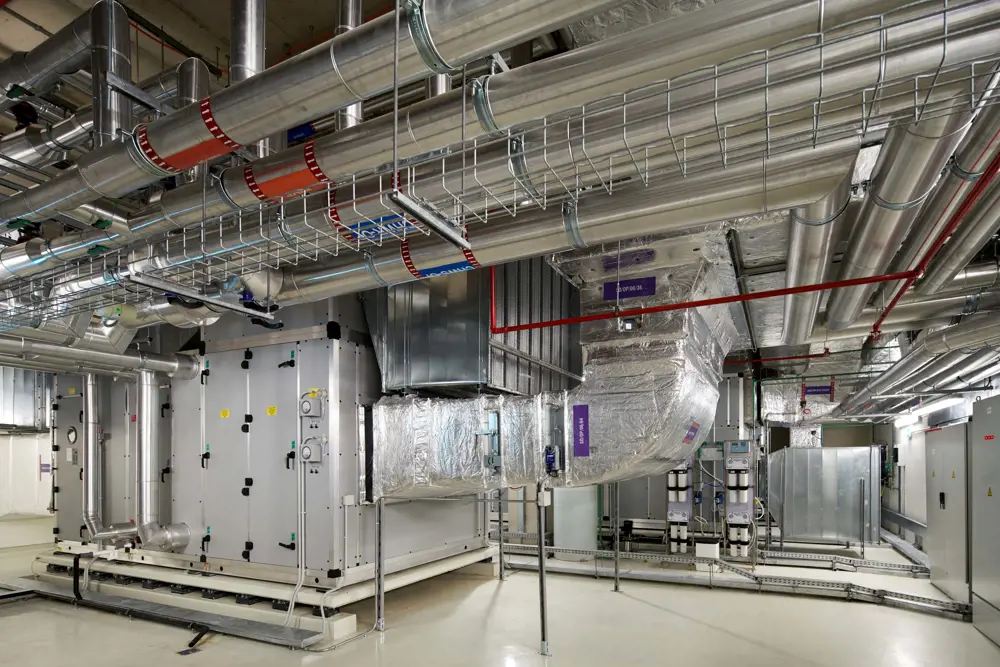
The air-handling unit supplying conditioned air into the opera theatre. The large ducts require lower air velocity and smaller fans, resulting in less noise close to the auditorium © Michel Denancé
The key to seismic protection for the building is the network of 320 pendulum base isolators underneath it, which reduce lateral seismic loads by 80%. Underlying soils are a mix of liquefiable sands and clays that can be softened. The building was effectively isolated from these by piles down to bedrock, but the artificial hill had to be protected with reinforced earth-retaining walls and localised stone columns sunk into the existing ground. A ‘buffer zone’ isolates the building from the hill.
The buildings themselves are much more conventional in design than the canopy, mainly using reinforced concrete, Greece’s most common building material. As part of the sustainability strategy, the buildings are, where possible, ‘layered’ like onion skins, with the most sensitive, carefully controlled environments (such as the rooms containing precious manuscripts) at the centre and rooms with greater tolerance to variations in temperature and humidity on the outside. Shade reduces solar gain, in particular the canopy over the opera house and carefully designed, retractable roller blinds on the glazed façades. The sloping park, which rises over the top of the library in the form of a grass roof, improves thermal insulation. Good insulation, low-energy lighting and extensive use of a highly efficient displacement ventilation system help to keep energy demand down. Air conditioning is still required, but its efficiency is increased by the use of heat recovery chillers. These recycle the heat rejected by the air conditioning to pre-heat domestic hot water from 20°C to 47°C, leaving conventional gas-fired heaters to do the rest.
All of the plant had to be hidden, with only limited space. Grey-water (all waste except from toilets) collection tanks are in the undercroft, and the large air intakes are through gratings in the park floor in the ‘buffer zone’ where the park joins the library roof. The chillers are discreetly located by the car park. In the vicinity of the opera house auditorium, the plant also had to be silent, leading to large low-ventilation ducts requiring relatively little fan power.
The net effect of all these measures is an estimated reduction in annual energy consumption of more than 40%, compared with a ‘standard’ building with an identical geometry and in the same location
The net effect of all these measures is an estimated reduction in annual energy consumption of more than 40%, compared with a ‘standard’ building with an identical geometry and in the same location. The 1,400-seat auditorium had a successful ‘test run’ in November 2016 when it hosted a speech by then President of the United States, Barack Obama, and the centre is due to be commissioned in stages over the early months of 2017, after which it will be handed over to the Greek State.
***
This article has been adapted from "Design-led innovation and sustainability", which originally appeared in the print edition of Ingenia 70 (March 2017).
Contributors
Hugh Ferguson
Author
Bruce Martin is an Associate Director at Expedition Engineering. He led the structural engineering design of the SNFCC. Bruce has a keen interest in elegant, innovative and practical engineering solutions that provide a clear benefit to the client.
Darren Barlow was the Project Director for the SNFCC project. He has over 25 years of experience in engineering design, encompassing an extensive range of general building services, environmental passive design techniques, and the application of renewable and low-energy technologies.
David McAllister was the Project Manager at Arup, leading the SNFCC project from concept through to construction. As the lead MEP (mechanical, electrical, plumbing) engineer, David guided coordination between disciplines and steered the sustainability strategy.was the Project Manager at Arup, leading the SNFCC project from concept through to construction. As the lead MEP (mechanical, electrical, plumbing) engineer, David guided coordination between disciplines and steered the sustainability strategy.
Keep up-to-date with Ingenia for free
SubscribeRelated content
Civil & structural

Building the Shard
The Shard is one of London's most iconic buildings. The tallest in Western Europe, it was designed by Italian architect Renzo Piano and dominates the city’s skyline. Ingenia spoke to John Parker, project director for structural engineers WSP, who outlined the engineering decisions made in building the enormous steel and glass structure.

The return of arched bridges
Arch bridges are strong, durable and require little maintenance. However, very few had been built since the early 1900s until the FlexiArch was developed and launched in 2007. Now, there has been a minor renaissance for this ancient form of construction.

Creating user-friendly buildings
For Michelle McDowell, a former Business Woman of the Year, a passion for joined-up design thinking and building information modelling with a user-friendly approach has enabled her to pioneer revolutionary changes in her field.

Troja Bridge
In November 2014, one of the world’s largest network arch bridges was officially opened in Prague. The UK may soon have its first network arch bridge if the go-ahead is given for a new rail project in Manchester.
Other content from Ingenia
Quick read
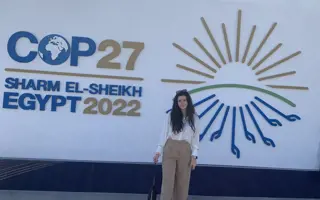
- Environment & sustainability
- Opinion
A young engineer’s perspective on the good, the bad and the ugly of COP27
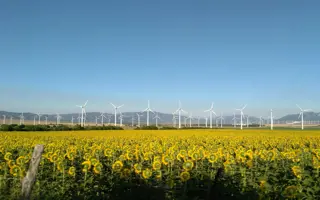
- Environment & sustainability
- Issue 95
How do we pay for net zero technologies?
Quick read
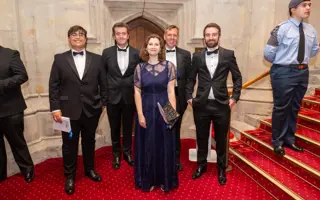
- Transport
- Mechanical
- How I got here
Electrifying trains and STEMAZING outreach
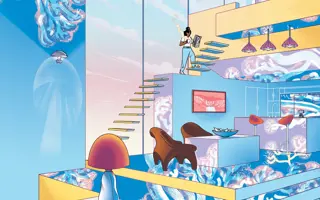
- Civil & structural
- Environment & sustainability
- Issue 95