
Compact atomic clocks
A brief history of timekeeping
⛵ Developing clocks for sea navigation to using atomic transitions to measure time
Timekeeping based on mechanical systems such as pendulums started to gain popularity in the 16th century. It was during this period that horologists first suggested that a precision clock might hold the key to the longitude problem by allowing mariners to accurately transfer ‘port time’ to sea. Clocks in those days tended to lose or gain time and did not operate reliably at sea or in different climatic conditions. The problem was solved by John Harrison’s revolutionary H3 sea clock, which removed the need for a pendulum. By the 20th century, more accurate clocks regulated by the steady vibration of quartz crystals began to replace mechanical devices. Lord Kelvin first introduced the idea of using atomic transitions to measure time in 1879, but it was not until the 1950s that the concept was practically demonstrated. The first atomic clock based on microwave atomic transitions was accurate to around one second in 300 years, making it 10 times more accurate than quartz-based clocks.
Researchers are now developing a new type of atomic clock based on optical atomic transitions that will allow time to be measured with a stability corresponding to one second in the age of the universe.
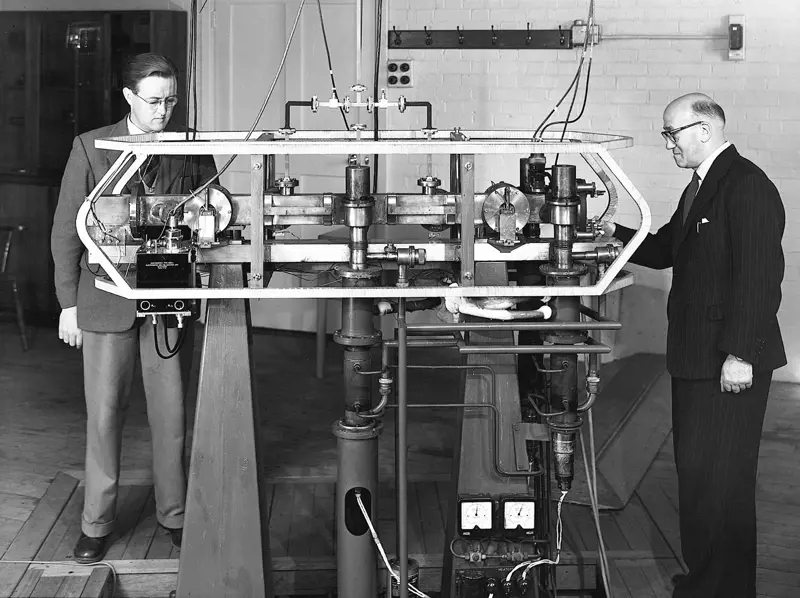
The first accurate atomic clock, a caesium standard based on a certain transition of the caesium-133 atom, was built by Louis Essen (pictured right) in 1955 at the National Physical Laboratory (NPL) in Middlesex. Calibration of the caesium standard atomic clock was carried out by the use of the astronomical time scale ephemeris time (ET). This led to the internationally agreed definition of a second being based on atomic time © National Physics Labaratory.
Our ability to determine time with ever-increasing precision underpins modern life. Global satellite navigation systems, the internet and utility infrastructures all rely on the accurate timing signals provided by atomic clocks, with transport infrastructures and logistics, safety-of-life critical systems and even global financial transactions increasingly demanding better timing accuracy.
When physicist Louis Essen demonstrated the world’s first accurate atomic clock in 1955 at the National Physical Laboratory (NPL) in the UK, it was not possible to foresee the impact that it would have on society. Since 1967, atomic clocks have defined our base unit of time, the second, as the duration of 9,192,631,770 periods of the radiation corresponding to the transition between the two hyperfine levels of the ground state of the caesium-133 atom. This was the first SI unit to be defined in terms of a fundamental unchanging quantity, paving the way for more robust definitions of the metre and other units.
Our ability to determine time with ever-increasing precision underpins modern life
The beat of Essen’s atomic clock was provided by microwave radiation whose frequency is adjusted to coincide with an atomic absorption, thereby exciting electrons in the atoms to move between particular discrete energy levels.
The state of the art in atomic timekeeping is being driven forward by institutions such as the NPL and similar labs such as the National Institute for Standards and Technology (NIST) in Colorado, US, with the latest atomic fountains offering stabilities of one second in more than 100 million years. These clocks, which work by launching a cloud of ultra-cold caesium atoms upwards and interrogating them using microwave radiation, require a vacuum vessel that stands nearly 3m tall in addition to extensive electronics racks and optoelectronic components to cool the atoms to micro-Kelvin temperatures.
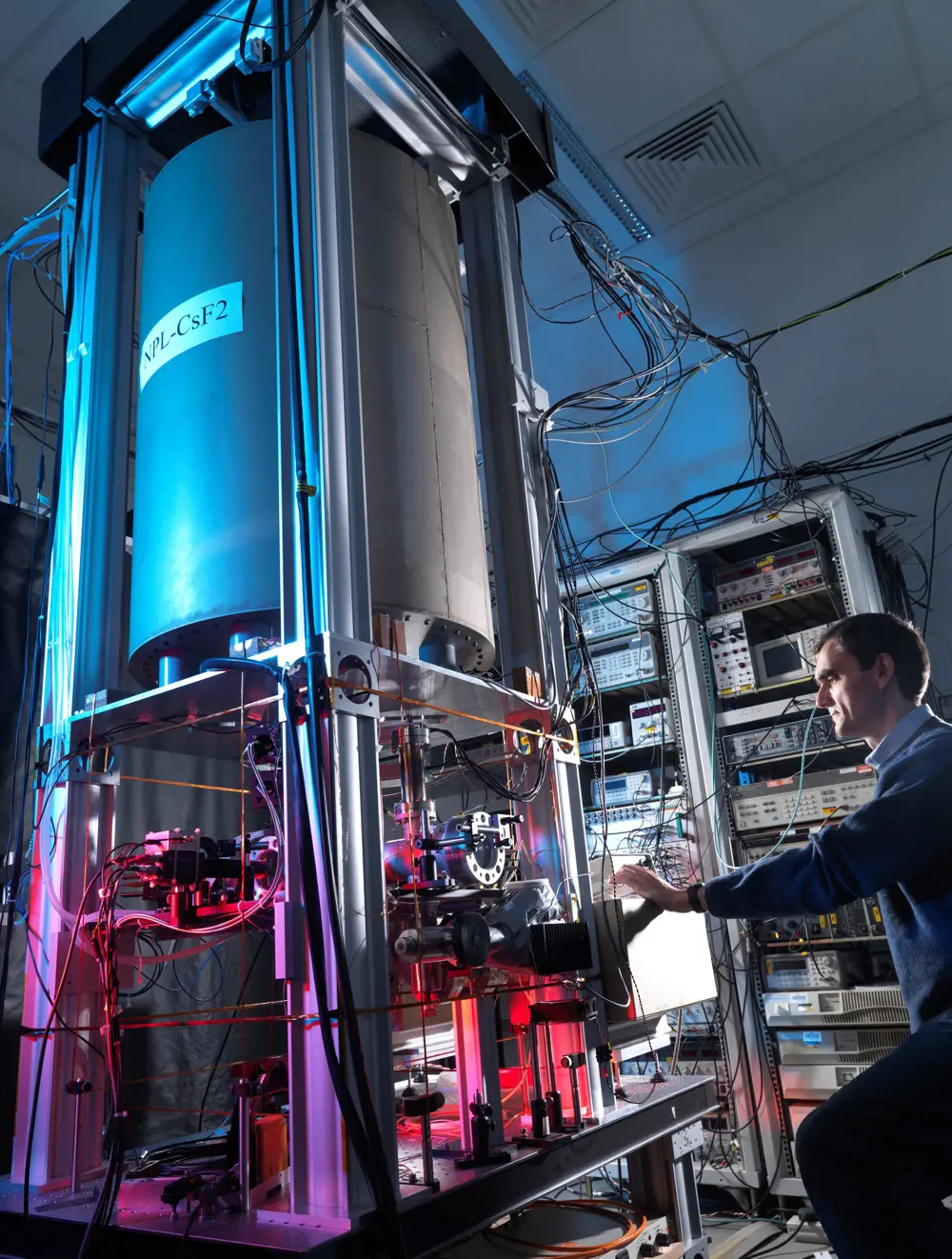
The National Physical Laboratory’s caesium fountain atomic clock ‘NPL-CsF2’ is a room-sized instrument that is used as the primary frequency standard for the measurement of time in the UK and contributes to International Atomic Time and Universal Coordinated Time © National Physical Laboratory
Smaller-scale clocks
A quiet revolution is also taking place in the area of ‘secondary’ industrial clocks and time standards. Lower accuracy commercial caesium and rubidium clocks are routinely used to disseminate time for a wide variety of applications. Typically housed within a 500 x 500 x 130 mm rack-mountable enclosure, they use thermal rather than cold atoms to achieve an accuracy of around one second in 30,000 years.
The last few years have seen the emergence of chip-scale atomic clocks that are much smaller and lighter, and require less power than their traditional counterparts. These devices incorporate a core ‘physics package’ of less than 1 cm3, with the complete clock system and associated electronics fitting inside the volume of a matchbox, and can provide accuracies in the region of one second in at least 300 years.
Such portable atomic clocks will benefit numerous civilian and military technologies, and will possibly lead to new ones. An atomic clock incorporated into a mobile phone, for instance, would allow greater connectivity to networks and larger data-handling rates by enabling data packets to be more tightly synchronised. Incorporated into a satnav device, chip-scale clocks would allow faster acquisition by the receiver of the Global Positioning System (GPS) signal and better interpolation during periods of signal drop-out, to provide more reliable navigation – see Applications for miniature atomic clocks.
An atomic clock incorporated into a mobile phone, for instance, would allow greater connectivity to networks and larger data-handling rates by enabling data packets to be more tightly synchronised
In fact, one of the key attractions of miniature atomic clocks is their ability to provide a local alternative to GPS satellites, which provide the atomic timing signals for most applications. A GPS outage – whether it is accidental, intentional from the use of GPS jamming hardware, or the result of natural phenomena such as enhanced solar flare activity – can have serious consequences. Ships crossing busy stretches of water such as the English Channel rely on GPS to stay in the correct lane, while certain telecommunications networks would drift and eventually seize up were they to be denied the GPS reference signals. Although additional global satellite navigation constellations such as Europe’s Galileo are soon to provide alternatives to the US-operated GPS, it is not possible to guarantee that satellite-based timing signals will always be available when required.
A recent Royal Academy of Engineering report, Global Navigation Space Systems: reliance and vulnerabilities, stated that chip-scale atomic clocks represent a “clear paradigm shift” in technological innovation – a view shared across wider UK government, industry and academia. Earlier this year, the UK Defence Science and Technology Laboratory (Dstl) issued a major call for proposals to solicit novel ideas from research and industry in the area of miniaturised atomic clocks, with an aim to reach new commercially viable clock and sensor systems for the UK’s defence and security sectors during the next five to ten years.
Applications for miniature atomic clocks
🛰️ From satellite navigation to financial transactions and communications
- Satellite navigation
Navigating in areas of intermittent GPS coverage would become easier if receivers were to contain a miniature atomic clock, which would allow local synchronisation to be maintained for longer periods and reacquisition of the GPS signal to be achieved in a couple of minutes rather than tens of minutes.
- Financial transactions
High-frequency trading requires a network to be synchronised with an accuracy of around 0.1 microseconds, otherwise it may appear that trades have occurred before they have actually been executed. Such networks are typically synchronised to GPS time and are hence susceptible to GPS outage.
- Flexible networks
Chip-scale clocks would allow powerful ad hoc communications networks to be established in the field for military purposes and enable data to be passed more reliably and securely via precision time stamping, data authentication and temporal windows.
- Secure communications
More generally, chip-scale clocks can potentially allow messages to be retrieved from data streams without the need for protocol ‘headers’ and ‘footers’, enabling messages to be transmitted with greater speed and security.
- Efficient communications
Chip-scale clocks allow the radio spectrum to be used more efficiently, for example via ultra-wideband spread-spectrum approaches, and help mitigate the high attendant data loss of undersea communications.
- Oil prospecting
The oil and gas industry is already using chip-scale atomic clocks to explore new fields and maximise recovery from existing fields, either in geophone and hydrophone sensor arrays for seismic exploration or in gradiometers and gravimeters for non-seismic exploration.
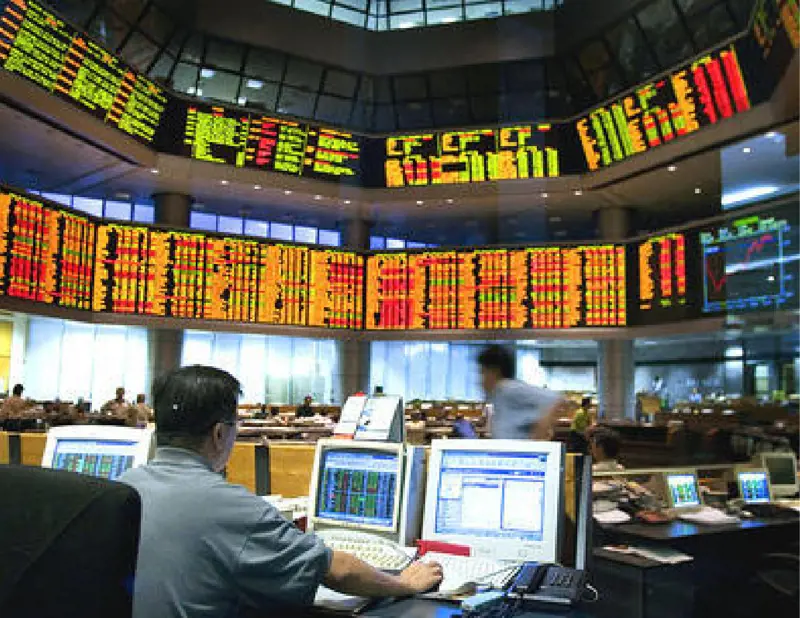
International stock exchanges don’t use the internet as a source for timing because of the risk of tampering by hackers and other malicious users. Such networks are typically synchronised to GPS time and are hence susceptible to GPS outage. Other non-GPS based networks use a precision time protocol with accuracy of the order of 10 microseconds
Commercial reality
Chip-scale atomic clocks (CSACs) have already become commercial reality in the US thanks to major investments by the Defence and Advanced Research Projects Agency (DARPA). Last year, the US firm Symmetricom launched a device with a volume of less than 17 cm3 that weighs only 35 g and consumes less than 120 mW of power, yet has a short-term stability corresponding to one second in 3,000 years. Other chip-scale clocks with comparable performances have been reported in the scientific literature but have not yet been designed with mass production in mind.
The principles underlying these miniature clocks are similar to those for their larger counterparts, but inevitable trade-offs against size, weight and power result in a less accurate device
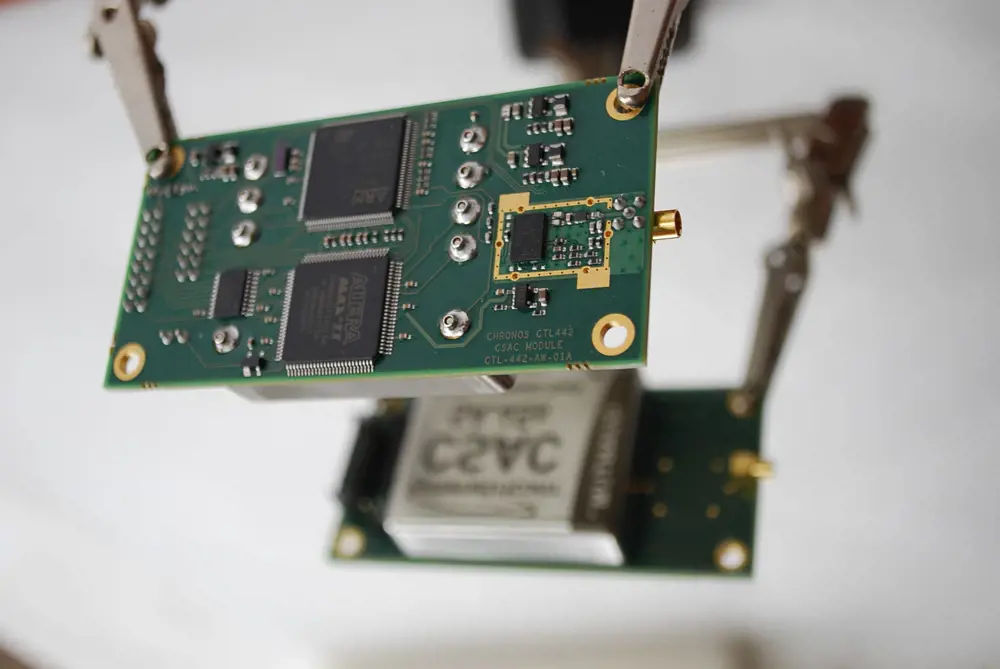
Synchronisation specialist Chronos Technology Ltd has integrated a chip-scale atomic clock with a GPS receiver to prolong the time during which the clock is synchronized with the satellite signals © Chronos Technology Limited
The UK firm Chronos recently integrated the Symmetricom CSAC into a printed circuit board along with a GPS receiver such that the satellite signal can be used to reference or ‘discipline’ the CSAC, and other suppliers are designing devices around the miniature unit. However, clocks need to be developed that offer even greater short-term stability so that the time spent waiting for devices to be synchronised can be reduced. Now that it has been shown that atomic clocks can be built small, the time and frequency community is being spurred on to create smaller, cheaper devices. The principles underlying these miniature clocks are similar to those for their larger counterparts, but inevitable trade-offs against size, weight and power result in a less accurate device.
A CSAC comprises three basic parts: a core atomic physics package, a microwave local oscillator and some electronic control circuitry. The physics package is typically a glass vapour cell filled with caesium vapour, in which caesium atoms are first excited by shining a laser through the cell with a wavelength tuned to almost match one of the atoms’ optical absorptions. When the microwave modulation frequency of the laser light is fully resonant with the caesium hyperfine clock transition, it changes the amount of light that is absorbed by the vapour. By monitoring the amount of light absorption using a sensitive photo-detector, the microwave resonance condition can be observed and this information used to lock the local oscillator to the hyperfine transition and thus derive a ‘one second’ tick.
The atomic processes taking place inside the vapour cell are based on a quantum mechanical phenomenon called coherent population trapping (CPT) – see Atomic interrogation. The concept dates back to the 1970s, but it took the confluence of various technologies to allow the breakthrough in CPT-based miniaturised atomic clocks as demonstrated by the Symmetricom device. For instance, micro-electromechanical (MEMS) fabrication methods are ideal for fabricating a suitably small vapour cell, while advances in semiconductor processing enable pinhead-sized lasers tailored to the wavelength needed to optically stimulate the caesium-133 vapour.

Atomic interrogation
⚛️ Coherent population trapping to provide a precise atomic reference
Coherent population trapping (CPT) describes a particular type of quantum mechanical interaction between a photon and a multi-level atomic structure. Caesium’s atomic structure can be pictured as a simple 3-level system comprising the two hyperfine ground states (0 and 1) and one excited state (2).
The laser light is split into two different optical frequencies or light fields using microwave modulation. If the difference in frequency between the two light fields exactly equals the splitting between the two ground states, the atoms can be pumped or ‘trapped’ into a coherent superposition of these two states (a quantum mechanical state where the atom is partly in level 0 and partly in level 1) via the coupling to level 2. A particular superposition of the ground states can therefore be made to appear ‘dark’ to the two laser light fields, since the probability of absorbing the laser photons approaches zero and the coherent dark state no longer couples to the excited state.
The reduced absorption gives rise to a spectrally-narrow increase in laser light transmitted through the atomic sample as the microwave resonance condition is met, which is then used to provide a precise atomic reference signal to which the approximately 9.2 GHz modulation frequency can be electronically stabilised.
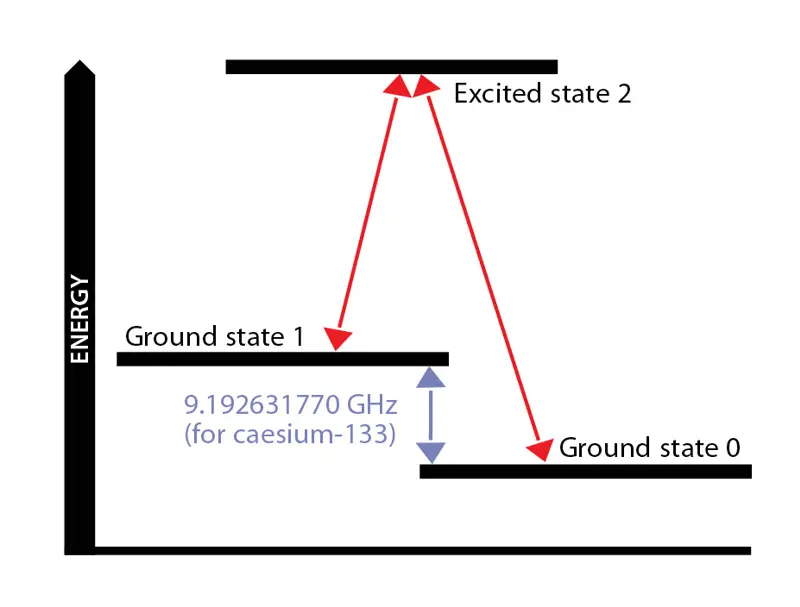
Physics package options
Two compact clock configurations show particular promise. In the first, which is already being employed in commercial devices, a cloud of caesium atoms in a vapour cell is heated to a few tens of degrees above ambient temperature and CPT employed to interrogate the system. The CPT technique avoids the need to directly probe the atoms with microwaves at 9.2 GHz. This is desirable because the ~ 3 cm wavelength of the microwave radiation limits the minimum size possible for a compact clock, whereas the CPT laser probe has a much smaller optical wavelength and beam waist. Chip-scale vapour cells generally comprise a MEMS cell with an internal dimension of around 100 µm, while other possibilities such as alkali-filled hollow-core fibres are also under investigation.
an increasing number of optical atomic clocks using laser-cooled trapped ions or atoms are demonstrating frequency uncertainties better than those achievable with the primary caesium fountain clocks
A second approach, aimed at improving on the precision of existing CSAC devices, makes use of laser-cooled trapped ions or atoms. These devices are more complex than thermal caesium architectures because they require additional laser systems to cool, prepare and probe the atoms or ions. However, since the cooled atoms or ions move at speeds of metres per second rather than several hundreds of metres per second as in the case of hot atoms, spectral (Doppler) broadening of the atomic reference transition due to the atoms’ thermal motion is significantly reduced. This gives rise to superior short-term stability over the non-cooled arrangement. In addition, ion-trap technology has advanced considerably in recent years, with photo-lithographic techniques allowing traps to be built into 350µm-thick silicon wafers, and is the focus for efforts at NPL and elsewhere to develop a high-accuracy miniaturised atomic clock that exploits either microwave or optical transitions.
Indeed, an increasing number of optical atomic clocks using laser cooled trapped ions or atoms are demonstrating frequency uncertainties better than those achievable with the primary caesium fountain clocks. The technology is likely to lead to a redefinition of the SI second within the next decade and has come about due to the availability of a device called a femtosecond frequency comb, which allows microwave frequencies to be directly derived from the optical clock frequency. Currently, the combs are tabletop devices, but there is significant research underway to develop micro-resonator versions that would lead to miniature optical clock variants in the medium term.
Atomic timekeeping
⏰ How international atomic and universal time is provided from the weighted average of over 200 clocks worldwide
International atomic time (TAI) and the leap-second-adjusted version, coordinated universal time (UTC), are provided by the Bureau Internationale des Poids et Mesures (BIPM) in Paris, from the weighted average of time kept by over 200 commercial atomic clocks (caesium clocks and hydrogen masers) from around 70 national laboratories worldwide.
Data files from these clocks are transmitted daily or weekly to the BIPM. The data include measured time offsets of each clock from that country’s UTC and regular time transfer data between the national timescales via two-way satellite transfer and GPS. The BIPM-constructed timescale is further ‘calibrated’ by data reported to BIPM for the same period from caesium fountain primary standards operated at a few of the national standards laboratories.
Cost considerations
The price of a miniature atomic clock currently starts at around $1,500, inhibiting the wide-scale civilian uptake of the technology. Military applications, meanwhile, tend to have specific requirements in terms of short-term frequency stability, operating temperature range and stability to vibrations and accelerations. It is highly unlikely that a single commercial product will meet the specific needs of every user.

A silica-on-silicon micro-trap, also shown with input laser light, could form the basis of a miniature atomic clock. The diode laser beams necessary for cooling and quantum state preparation are launched from optical fibres through a window above the wafer trap © National Physical Laboratory
Current and prior investment in atomic clock research and related quantum technologies has provided a strong foundation on which to develop new compact clock technologies, but the cost of designing and manufacturing the physics package represents a commercial barrier. This cold atom technology is currently laboratory-based with a high infrastructure overhead, for example, with the need to laser cool atoms or ions to micro-Kelvin temperatures in very high vacuum enclosures. The challenge is to miniaturise this ancillary infrastructure to realise a compact system solution.
Ideally, a coordinated programme across government, academia and industry is needed to explore miniature atomic and quantum configurations that can lead to versatile and compact positioning, navigation and timing instrumentation.
Peering through a crystal ball, the capabilities afforded by miniaturised atomic clocks in combination with quantum inertial sensors that measure accumulated rotations and/or gravity gradients open a new world of opportunities. There could come a time when a fully autonomous underwater vehicle could circumnavigate the world with a precision of a few centimetres for an extended period of years, without the need for satellite-based timing signals.
Over the past two years, the UK Ministry of Defence and Dstl, together with NPL, have worked to enable a coordinated compact-clock research programme. Successful outcomes will lead to atomic timekeepers and other quantum sensors that will suit a range of applications in the aerospace, defence and security and environmental sectors.
For more about this programme see: www.npl.co.uk/science-technology/time-frequency/
***
This article has been adapted from "Compact atomic clocks", which originally appeared in the print edition of Ingenia 65 (December 2015).
Contributors
Sir Peter Knight FRS is the immediate past President of the Institute of Physics and Emeritus Professor of Quantum Optics at Imperial College London, where his research interests include quantum information processing and strong field physics. He is a Fellow of the Royal Society, and of the Institute of Physics and of the Optical Society of America (where he was also President).
Dr John Christensen FIET is Group Leader of the Time and Frequency group at NPL. He worked within government for 31 years, providing technical assurance to, and governance of, a variety of pure and applied research programmes in the areas of components, sensors, guidance and navigation. He is working on engineering applications leading to the miniaturisation atomic clocks and quantum inertial sensors.
Professor Patrick Gill FInstP is a Senior Fellow at NPL and head of the Time and Frequency group there. He is concerned with the UK realisation of the primary SI unit of time, the second, and research into future optical clocks. Professor Gill joined NPL in 1975 after completing his DPhil in laser research at the University of Oxford.
Keep up-to-date with Ingenia for free
SubscribeRelated content
Software & computer science
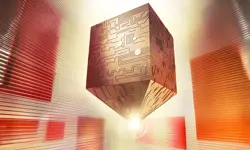
Pushing the barriers to model complex processes
In 2007, Imperial College London spinout Process Systems Enterprise Ltd won the MacRobert Award for its gPROMS (general-purpose PROcess Modelling System) software. Costas Pantelides and Mark Matzopoulos, two of the key people behind the success of gPROMS, tell how they created a way in which engineers can harness physics, chemistry and engineering knowledge within a framework that solves highly complex mathematical problems.
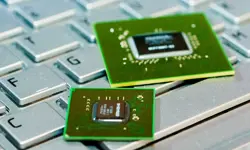
The rise and rise of GPUs
The technology used to bring 3D video games to the personal computer and to the mobile phone is to take on more computing duties. How have UK companies such as ARM and ImaginationTechnologies contributed to the movement?
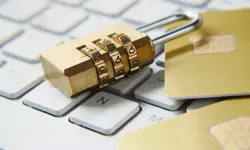
EU clarifies the European parameters of data protection
The European Union’s General Data Protection Regulation, due for adoption this year, is intended to harmonise data protection laws across the EU. What are the engineering implications and legal ramifications of the new regulatory regime?

Evolving the internet
He may have given the world the technology that speeded up the internet, but in his next move, Professor Nick McKeown FREng plans to replace those networks he helped create.
Other content from Ingenia
Quick read
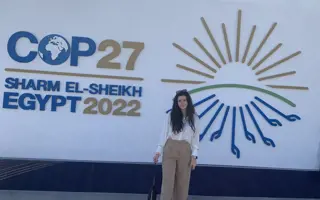
- Environment & sustainability
- Opinion
A young engineer’s perspective on the good, the bad and the ugly of COP27
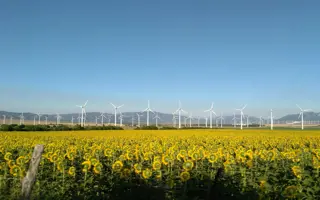
- Environment & sustainability
- Issue 95
How do we pay for net zero technologies?
Quick read
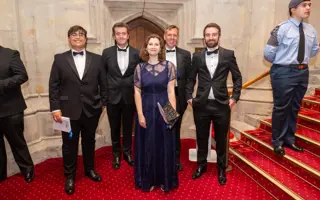
- Transport
- Mechanical
- How I got here
Electrifying trains and STEMAZING outreach
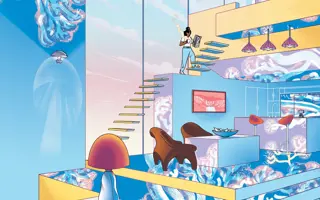
- Civil & structural
- Environment & sustainability
- Issue 95