
3D printing a bridge with a twin
Did you know?
👯♀️ Why are engineers twinning bridges?
- Sensors are being embedded into structures new and old, from tunnels to bridges, so that engineers can monitor whether repairs are needed using so-called ‘digital twins’
- Data from these sensors could help engineers design smarter structures, for example with less material to save CO₂ emissions
- A canal bridge in Amsterdam – the first ever 3D-printed steel bridge – has had a digital twin created, so engineers can demonstrate what they are capable of
In Amsterdam’s red light district, a bridge unlike any other stretches across the Oudezijds Achterburgwal canal. It’s strikingly different from the brick arches elsewhere in the city, with bicycles locked to metal balustrades. But it’s not just its appearance that’s different.
This is the world’s first 3D-printed steel bridge, and it is outfitted with over 100 sensors that measure loads applied to the bridge, wind, air quality, and much more. This makes it more than a footbridge, and more than a design object. It is a living laboratory, a testbed for digital twins (see ‘Creating a virtual replica’, Ingenia 87) of public infrastructure.
The data collected from its sensors are sent to the Alan Turing Institute in London, where it will feed into a continuously updated digital model, or ‘digital twin’, of the bridge. Engineers will use this to monitor it in real time, while the City of Amsterdam will use it to analyse pedestrian behaviour. But importantly, it will also fuel research into digital twins of structures that will help optimise future designs, enabling engineers to reduce material use and, as a result, carbon emissions.
The 3D-printed bridge will fuel research into digital twins of structures that will help optimise future designs, enabling engineers to reduce material use and, as a result, carbon emissions.
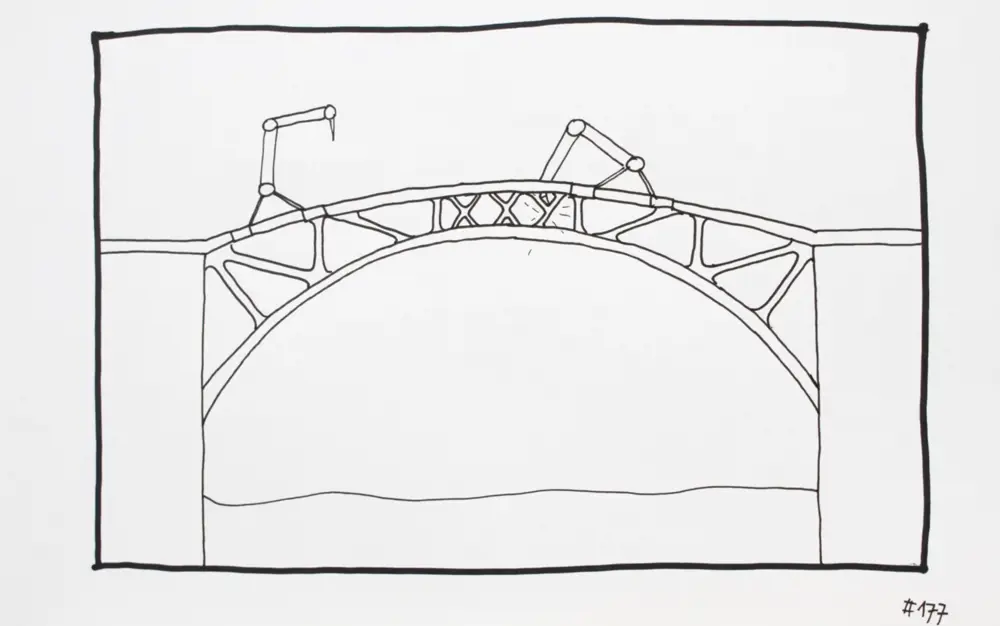
The first design for the bridge was a skeletal structure, rejected by the Municipality as the lower limbs could be struck by the heavy boats using the canal © MX3D
An elaborate publicity stunt
The origins of this bridge lie within a small creative design studio in Amsterdam, Joris Laarman Lab, headed by designer and artist Joris Laarman. In about 2014, excited by opportunities presented by emerging technologies, the team decided to develop designs in 3D-printed stainless steel. This presented an immediate challenge: no-one had before produced large steel objects using 3D printing or additive manufacturing.
The process requires molten metal to be deposited in multiple layers. At the time, there were already tools for metal inert gas (MIG) welding. In this arc welding process, a continuous solid wire – usually 1.2 millimetre in diameter – is electrically heated and fed from a welding gun. There were also robots on which the tools could be mounted. However, no-one had used robots with MIG welding. Robots were generally used for repetitive ‘pick and place’ tasks, rather than complex welding control.
The main challenge was to create software to control such uniquely programmed toolpaths. So, the team formed a startup, MX3D, invested in equipment and started to experiment.
MX3D recognised that it needed publicity to get further support. So, the team started producing eye-catching objects, including chairs and bicycles. But from the start, they realised that a steel bridge would put them on the map. And where better to get noticed than across the Oudezijds Achterburgwal, one of Amsterdam’s oldest canals, where millions would see it?
Even before a design was firmed up, MX3D’s efforts at publicity proved extraordinarily successful. A number of international bodies came to join the project as partners, including software company Autodesk and steel manufacturer ArcelorMittal. The structural engineers at Arup helped to create a new design. Universities also became involved, including Imperial College London for testing, and the Alan Turing Institute for creating a digital twin.
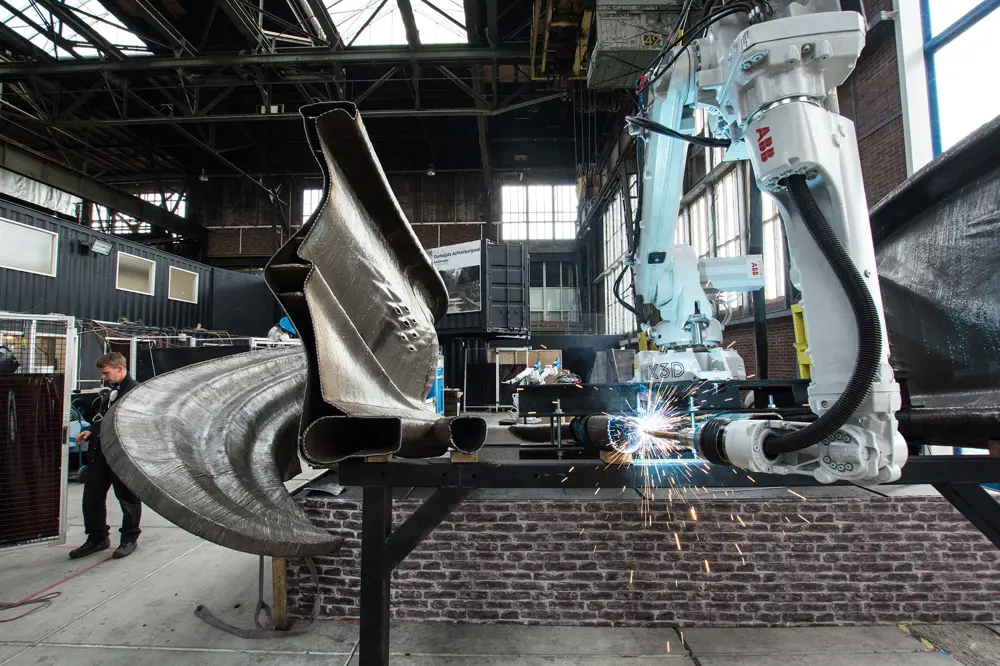
Printing of the bridge underway at MX3D’s workshop in Amsterdam © Olivier de Gruijter
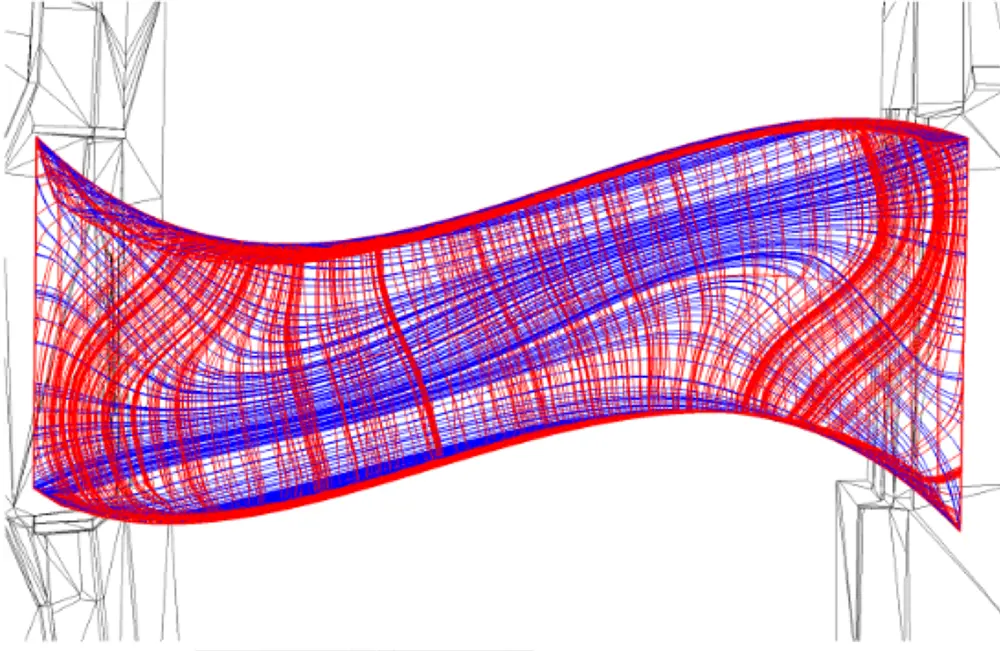
The stress analysis performed by Arup © Joris Laarman Lab
Printed by robots
The team worked with Arup to create a new design, starting with a simple U-beam section to span the 8.5-metre-wide canal, on Arup’s advice. Arup added design loads to a finite element model of the bridge and presented pictures of the stress patterns. These inspired Laarman to adjust the design.
He used them to guide the structural appearance – to add extra material thickness where more strength was needed or subtract material in places with lower stress. The materials and design fell outside the normal design codes that govern bridge structures, so universities including Imperial College London undertook a major programme of testing, starting with small samples and concluding with a full-scale load test of the completed bridge. These confirmed that the material’s characteristics were similar to conventional stainless steel.
Four robots, each with six-axis arms, then printed the full 6,000-kilogram bridge in six months in MX3D’s workshop. A full-scale load test, with the maximum design load of 20 tonnes, then confirmed the bridge’s overall strength.
To simplify construction, the team printed the bridge in several parts and transported it by road and boat from MX3D’s workshop. The central structural section spans the canal, with paving added later, while the ornate flourishes at either end were manufactured separately and welded on.

The central structural section of the bridge being transported to its final location © Merlin Moritz
Digital twinning
Meanwhile, the Alan Turing Institute in London, the UK’s national institute for data science and artificial intelligence, and Professor Mark Girolami FREng, its Chief Scientist, became interested. It adopted the bridge as a testbed and demonstrator for digital twins in construction as part of its datacentric engineering programme.
The bridge has been fitted with more than 100 sensors that measure strain, displacement and vibration, people-flow and the loads they apply on the bridge. The sensors also measure environmental conditions, including issues relating to natural corrosion, wind, thermal, light, and air quality.
The data from the bridge's sensors is transmitted to a control centre at the Turing Institute to help engineers to monitor and maintain the bridge over time. It will also fuel more research into machine learning, data analytics and management, and optimised design, to show what can be achieved with digital twins.
As an example, measuring ‘natural corrosion’ means that if in several years’ time the strength of this new material started to deteriorate, the digital twin would spot this long before there were any visible indicators. Some others (such as wind) are less relevant to this bridge, but could be very important on a suspension bridge, so are more significant for this bridge as a demonstrator for digital twinning on structures in general.
The data from these sensors is sent to servers in a basement room adjacent to the bridge, before being transmitted directly to a control centre at the Turing Institute. It will not only help engineers to monitor and maintain the bridge over time, but will also fuel more research into machine learning, data analytics and management, and optimised design. This will help show what can be achieved with digital twins.
Bringing data to the forefront
The work of the Turing Institute in creating a digital twin for the Amsterdam bridge is an important steppingstone in a career-long campaign by Lord Robert Mair CBE FREng FRS, Emeritus Professor of Civil Engineering at the University of Cambridge, to persuade members of his profession to measure the performance of what they create. His work is being continued by Professor Girolami, who is successor to his chair at Cambridge.
Lord Mair believes that civil engineering is a conservative profession, and that other sectors – notably aeronautics, automotive engineering and electronics – are upgrading and improving, with leaner and smarter design, because they measure what they do. Design, he believes, should be performance-based: measure what you build, and use the data to maintain existing structures and improve the design of new ones.
“I’m a huge fan of civil engineering,” he says, “but we are ... doing the same old designs, same old codes of practice, same old methods, which were all founded around uncertainty – because we don’t know how something actually works.”
Decades ago, he was consulted about London’s Tube tunnels. He was asked about the life expectancy of the 100-year-old Northern Line, and concern about movement of the 1970s flexible tunnel linings at Bond Street. In both cases, his advice was to insert strain gauges to measure movement over time. If there was no movement, then ‘if it ain’t broke don’t fix it’, but keep monitoring. If movement was detected, then the scale and speed of the movement would indicate what needed to be done.
Later, on his advice, strain gauges were cast into the concrete tunnel segments of London’s new power tunnels before construction. This meant that performance of the tunnels could be measured over decades to come – a world first.
He also established the Cambridge Centre for Smart Infrastructure and Construction (CSIC), largely to persuade the industry to adopt such measurement and monitoring as a norm. At the same time, there were major developments in data, wireless technology and fibre optics, all of which helped with improving measurement.
The adoption of modular construction has also furthered the cause. Since key elements are fabricated in a factory rather than on site, sensors can easily be embedded in the structure (as with the Thames Power Tunnels). This is more reliable than adding them on later.
Strategic maintenance, future design
A major advantage of closely measuring structural performance is that it enables more strategic maintenance. Network Rail has a major continuous programme of visual inspection and maintenance of its vast infrastructure. It asked CSIC engineers to install 200 fibre-optic strain gauges into two new bridges in Staffordshire to detect the real stresses as trains pass over, as opposed to those assumed by the designers (Ingenia 87).
The first result was to reveal that the bridges are much stronger than needed to carry the loads required. As a result, future bridges could be designed and built with less material – at a lower environmental and economic cost. Longer term, the sensors will continuously feed back data on the bridges’ performance, revealing the need for repairs.
CSIC was also asked to look at a large Victorian railway viaduct through Leeds, which Network Rail was planning to demolish and replace. Installing strain gauges revealed that the bridge was actually performing remarkably well under the loading from highspeed trains, despite alarming cracks in the masonry. This £30,000 investment saved tens of millions of pounds for replacement, and the sensors remain to detect any future deterioration. Repeated over Network Rail’s whole network of structures, this approach could greatly reduce the amount of visual inspection needed and help to prioritise where maintenance is most needed.
The approach can also inform future design, so that engineers can reduce CO2 emissions associated with construction projects, along with overall costs. In one example that hinted at what was to come, CSIC engineers inserted fibreoptic strain gauges into the huge circular concrete shafts for both Crossrail and Thames Tideway in London during construction. The shafts were designed as a series of large retaining walls, crammed full of steel reinforcement to resist bending from the pressure of the surrounding earth.
The sensors revealed that the bending was actually minimal, meaning future such shafts can be designed with far less reinforcement, and substantial cost (and CO2) savings. Similarly, monitoring Crossrail tunnels at Liverpool Street station showed that far more reinforcement than necessary was used to strengthen tunnel linings at junctions with crossover passages – with a potential saving of £1 million per station.
Lack of knowledge about how structures actually behave has meant that current standards and Codes of Practice embody large factors of safety to cover uncertainty. As the availability of data improves our understanding of real structural behaviour, some of these standards could potentially be relaxed. Of course, for very good reasons, changing Codes of Practice takes time and should be proceeded with cautiously. Lord Mair can see, for example, piles being 10% less thick and retaining walls up to 20% less thick, with huge savings. The data will also assist the creation of codes and standards for new materials, such as 3D-printed steel.
Digital twins are an extension of this philosophy, now made possible by technological developments such as the ability to store and analyse vast amounts of data. For every future infrastructure project, Lord Mair and Professor Girolami would like to see a digital twin created in parallel with the design – and not a spade put in the ground until the digital twin is complete. Then, data from the digital twin could inform the construction process, while data fed back from sensors during construction could help refine the digital twin.
For every future infrastructure project, Lord Mair and Professor Girolami would like to see a digital twin created in parallel with the design – and not a spade put in the ground until the digital twin is complete. Then, data from the digital twin could inform the construction process, while data fed back from sensors during construction could help refine the digital twin.
Currently, a typical project may be designed and supervised by a consulting engineer and handed over to the client on completion, together with a ‘good luck’ message. Mair would like to see the digital twin handed over as well, continuously monitoring the structure in use and providing ‘red light’ warnings if anything should go awry. The Amsterdam 3D-printed bridge is perhaps the most highly developed example so far. Then, the use of the digital twin could go further. Professor Girolami likes to quote the English statistician George Box who said, ‘all models are wrong’. This is because of the inexact assumptions within them, and applies even to sophisticated finite-element models (FEMs) used to design the most complex structures.
If real-time data from the bridge was fed into the FEM used for design, the fidelity of the model could be improved. It would not be as ‘wrong’ as it was at the start, and it could be trusted more. For example, it could be used to predict how a railway bridge would perform if an overweight train passed over it. And by extension, the FEMs for designing future structures could then be tuned to make them more accurate predictors.
This in turn enables what is called generative design. By establishing a set of criteria and design constraints and feeding in the data given out by the digital twin, engineers can then generate many designs that meet these constraints and criteria. This would be a major step forward for structural engineering.
Professor Girolami believes this datacentric multidisciplinary approach to capturing the bridge’s data marks a step-change in the way bridges are designed, constructed and managed.
***
Hugh Ferguson thanks Gijs van der Velden, CEO of MX3D; Professor Mark Girolami FREng, Professor of Civil Engineering at the University of Cambridge and Chief Scientist at the Alan Turing Institute (and formerly Professor of Statistics in the mathematics department at Imperial College London); Professor Lord Robert Mair CBE FREng FRS, Emeritus Professor of Civil Engineering at the University of Cambridge and founder of the Cambridge Centre for Smart Infrastructure and Construction; and Mathew Vola, Project Director in Arup’s Amsterdam office, for their help in preparing this article.
***
This article has been adapted from "3D printing a bridge with a twin", which originally appeared in the print edition of Ingenia 94 (March 2023).
***
More on the story
🌉 A bridge very far: how they built the world's longest suspension bridge a year early
Contributors
Hugh Ferguson
Author
Keep up-to-date with Ingenia for free
SubscribeRelated content
Civil & structural

Building the Shard
The Shard is one of London's most iconic buildings. The tallest in Western Europe, it was designed by Italian architect Renzo Piano and dominates the city’s skyline. Ingenia spoke to John Parker, project director for structural engineers WSP, who outlined the engineering decisions made in building the enormous steel and glass structure.

The return of arched bridges
Arch bridges are strong, durable and require little maintenance. However, very few had been built since the early 1900s until the FlexiArch was developed and launched in 2007. Now, there has been a minor renaissance for this ancient form of construction.

Creating user-friendly buildings
For Michelle McDowell, a former Business Woman of the Year, a passion for joined-up design thinking and building information modelling with a user-friendly approach has enabled her to pioneer revolutionary changes in her field.

Troja Bridge
In November 2014, one of the world’s largest network arch bridges was officially opened in Prague. The UK may soon have its first network arch bridge if the go-ahead is given for a new rail project in Manchester.
Other content from Ingenia
Quick read
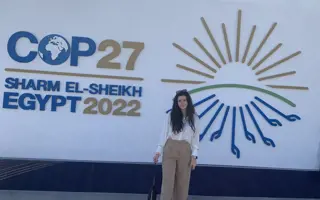
- Environment & sustainability
- Opinion
A young engineer’s perspective on the good, the bad and the ugly of COP27
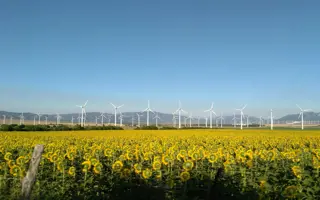
- Environment & sustainability
- Issue 95
How do we pay for net zero technologies?
Quick read
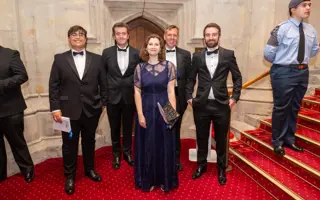
- Transport
- Mechanical
- How I got here
Electrifying trains and STEMAZING outreach
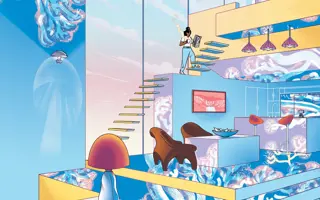
- Civil & structural
- Environment & sustainability
- Issue 95