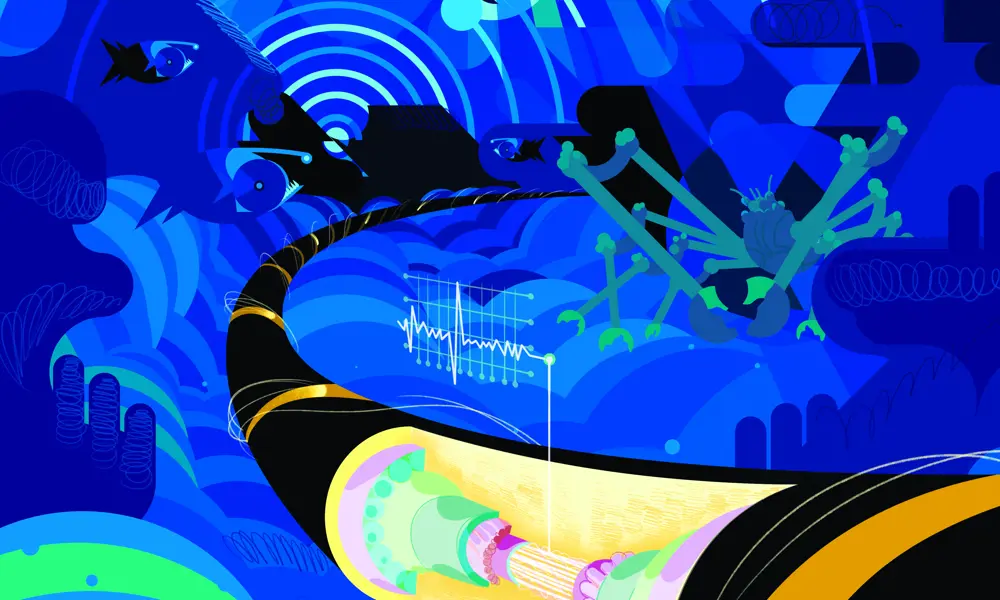
Undersea information sharing
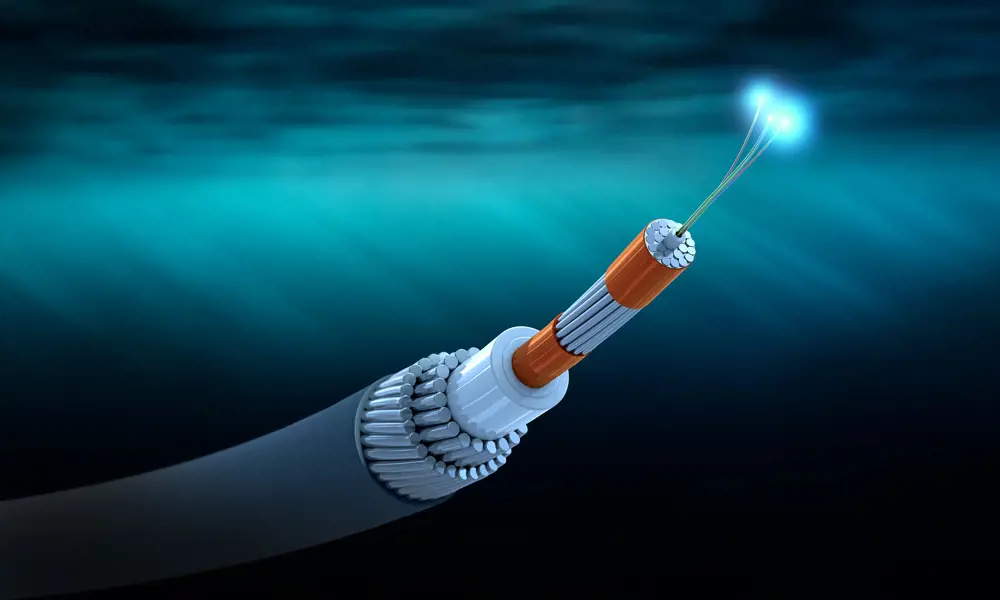
Our growing reliance on mobile and digital technologies is driving an almost insatiable demand for data. During the COVID-19 pandemic, people started increasingly working from home and kept in touch with colleagues, friends and family via video calling. This, coupled with widespread use of streaming services, 5G mobile networks and online gaming, saw global internet usage rise by almost 30% a year between 2017 and 2021. And these numbers show no sign of slowing down: new applications and digital services are released every year, millions more people are becoming connected to the internet, and we are living more of our lives online.
Network operators are responding to this surging demand by extending their fibre networks, providing dedicated, high-speed connections to homes and businesses to support data-hungry services such as video platforms and cloud computing. This urgent need for extra capacity and ever-faster speeds also extends to the long-distance optical cables that criss-cross the world’s oceans, laid on the seabed. Between them, these subsea cables carry some 99% of the world’s telecommunications traffic.
Between them, these subsea cables carry some 99% of the world’s telecommunications traffic.
As a result, there has been an increase in installations of these cables across all major routes. Most new deployments are funded by organisations such as Google, Amazon, Microsoft, and Meta, which together account for about two-thirds of all internet traffic – and an astonishing 90% on the busiest transatlantic links. According to figures from industry analyst TeleGeography, over $10 billion worth of new subsea cables will enter service between 2022 and 2024, and demand on these links is expected to increase at a compound annual rate of 30% to 50% between 2021 and 2028.
Each new deployment costs hundreds of millions of pounds, so engineers need to design submarine cable systems with longevity in mind. Intense technical innovation has focused on increasing the capacity to send more information over each subsea link, with many cables now carrying more than one hundred terabits per second (Tbit/s). The current record is held by Google Cloud’s Dunant cable, which supports 250 Tbit/s – equivalent to sending the full digitised collection of the world’s largest library, the US Library of Congress, three times every second – over a 6,600-kilometre link connecting Virginia Beach in the US with Saint-Hilaire-de-Riez in France.
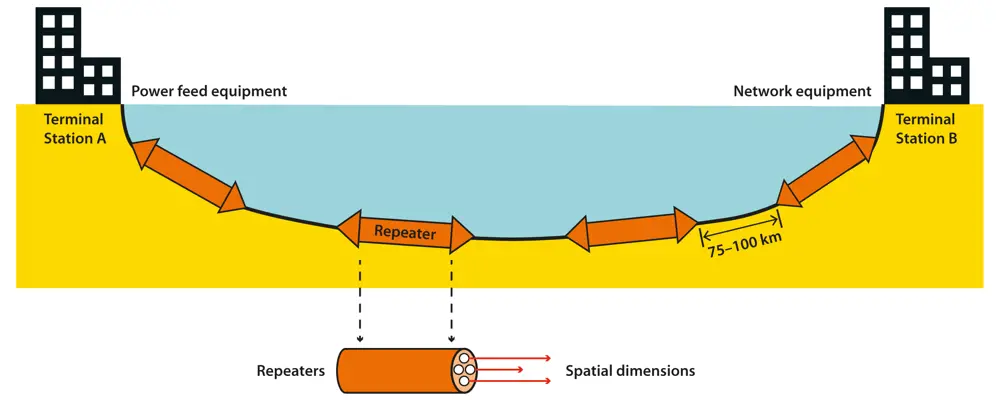
The cable system
A submarine optical cable provides a communications link between two or more landing points. At each end of the cable, a landing station houses the optical transmission equipment that sends and receives the optical signals, along with a power-feeding system that supplies a continuous direct current to a series of optical repeaters installed along the length the cable. In conventional systems the repeaters contain a dedicated optical amplifier for each fibre, but the number of fibres that can be supported is then limited by the amount of power that can be delivered from the landing stations. In contrast, an SDM system shares the available power between the optical channels, making it possible to increase the number of fibres and boost the data-carrying capacity of the cable.
Hungry for data
Such capacity increases have generally been the result of ongoing advances in the optical and electronic equipment installed at each end of the submarine cable, which have boosted the amount of data that can be sent through each optical fibre within the cable. In particular, the introduction of so-called coherent transmission technologies has made it possible to send and receive the complete optical signal rather than just the intensity of the beam, delivering a 10-fold increase in capacity since 2010.
However, packing more data into individual optical channels also means that each one needs a greater power supply. For subsea links, that power can only be delivered at the landing stations at either end of the cable, with periodic repeaters installed along its length to maintain the signal strength over intercontinental distances.
This challenge places a practical limit on the number of fibres that can be supported within a subsea link, with even the most advanced systems only able to carry data over 16 optical fibres. In telecoms networks these individual fibres are traditionally formed into pairs, with each fibre pair carrying two-way communications traffic. “The capacity we can achieve is limited by the power of the subsea cable,” explains Jeremie Renaudier, a lead researcher at Nokia Bell Labs in France. “We cannot increase the number of fibre pairs in these systems without having enough power to optimise the capacity and performance of each one.”
Creation of the Dunant cable marked a change in strategy. A complete redesign of the system architecture meant that the fibre pairs could share the available power, rather than each one having its own dedicated power source. This power-sharing scheme, called space-division multiplexing (SDM), enabled Dunant to become the first long-distance subsea cable to support 12 fibre pairs – rather than eight – delivering a 40% increase in capacity. “The system is suboptimal on a per-fibre basis, but distributing the power increases the capacity of the whole cable,” explains Renaudier.
The success of the approach means that submarine links supporting 16 optical channels are already coming into operation, while two long-distance SDM systems capable of transmitting about 500 Tbits/s over 24 fibre pairs will soon enter service: the 2,600-kilometre Confluence-1 system that will connect Miami with New York in 2023, and the EU-supported 8,800-kilometre Medusa cable that is due to link Europe with North Africa in 2024.
The capacity we can achieve is limited by the power of the subsea cable.
Jeremie Renaudier, a lead researcher at Nokia Bell Labs in France
A tight squeeze
However, the demanding subsea environment places other practical limits on the number of fibre pairs that even an SDM system can support. At the most fundamental level, all of the fibre pairs must fit into a standard-sized cable, which is typically 17 or 24 millimetres across. “These cables are made from expensive materials, and so increasing the cable diameter adds significantly more cost into the system,” explains Fatih Yaman, a senior researcher at NEC Labs in the US.
As a result, current research is focused on squeezing more fibre pairs inside a standard-sized cable, with the limit expected to be about 32 or possibly even 48. At the same time, cable companies such as NEC are already preparing for a future when it will no longer be economically viable to add more fibres into the cable, and Yaman believes that the most obvious way forward is to introduce more sophisticated fibre technologies that support multiple optical cores. “Multicore fibre (MCF) is the only way to scale the number of optical channels without increasing the cable diameter,” he says.
Indeed, NEC engineers have been working with fibre manufacturer Sumitomo Electric to develop a prototype submarine cable based on four-core fibre, with each one supporting 16 fibre pairs. In laboratory tests simulating real-world conditions, a 3,000-kilometre cable delivered a capacity of more than 1.7 petabits per second, and in 2021 the company completed the first field trials of its prototype design.
While MCF could become technically feasible, it is currently much more expensive to produce than standard fibre. “It’s not yet clear whether it’s a technical limitation or mainly a question of manufacturing volume,” says Yaman. “The issue for the industry right now is how fast and how far the costs come down as volumes increase.”
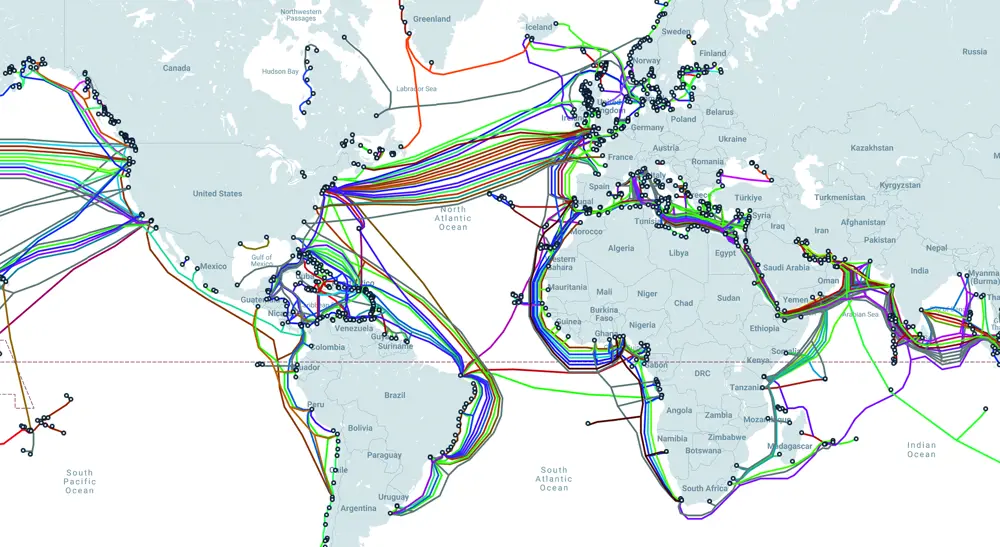
Undersea sensors
While the telecoms industry is focusing on maximising the capacity of these networks, environmental engineers are hoping to unlock the optical information transmitted by underwater links to create a global network of geophysical sensors. Any underwater disturbance can affect an optical signal as it travels along a fibre, which scientists have already exploited to detect the vibrations caused by seismic and storm activity. Researchers in Norway have even shown that a submarine cable can pick up the sound of singing whales, offering an effective way to track their migration along the Norwegian coast.
Several different sensing modalities have been developed and tested in recent years. The Norwegian whale trackers used a technique called distributed acoustic sensing (DAS), which listens for underwater sounds by sending an optical pulse through a fibre and then analysing the signal that is reflected back from different points along the cable. While this technique can pinpoint the source of the sound to within a few metres, its range is limited to a few hundred kilometres from the shoreline.
Other approaches unpack the information in an optical signal after it has travelled through the length of the cable – making it possible to detect vibrations and acoustic disturbances over much longer distances. In 2018 a team led by Giuseppe Marra, Principal Scientist in the National Physical Laboratory’s (NPL) time and frequency department, demonstrated a technique for detecting earthquakes using standard communications cables. By sending light from an ultrastable laser through a terrestrial link a few hundred kilometres long, the team could measure changes in the optical signal that were caused by seismic events up to 18,500 kilometres away.
Then, in 2021, a collaboration between the California Institute of Technology and Google showed that regular telecommunications traffic could detect earthquakes as well as storm-driven ocean swells. Using data collected over a 10,000-kilometre submarine cable, the team recorded about 30 storm-swell events and multiple moderate-to-large earthquakes over a period of nine months, including the magnitude 7.4 earthquake event that happened near Oaxaca, Mexico, in June 2020.
While promising, the downside of these techniques is that they measure the cumulative effect on the optical signal over the entire length of the cable. That makes it difficult to locate the source of the disturbance, such as the epicentre of an earthquake, without further information from other cables or monitoring systems. Noise also builds up along the length of the fibre, preventing the detection of smaller environmental events.
In 2022 the NPL team addressed this issue by adapting its technique to measure the change in optical signal between the repeaters on a 6,000 kilometre section of a cable, rather than over the whole length. “With our previous work the cable acted as a single sensor,” explains Marra. “In this new study we have exploited the technology inside the optical repeaters to transform the cable into an array of sensors, where each sensor is now the span between each repeater.”
The team tested its updated scheme over several months using a live communications link between the UK and Canada. As well as detecting earthquakes, the team could locate the source of individual events and track their evolution in both space and time. The measurements also revealed the effects of ocean currents, as well as periodic disturbances that the team attributes to tidal activity. “An integrated view over the whole cable picks up lots of events, but it’s difficult to make sense of them,” comments Marra. “With this technique we can pick out features in specific sections of the cable, which makes it a much more powerful tool.”
Marra is confident that the team’s experimental set-up could easily be engineered for use with other submarine cables, since no changes are needed to the existing underwater infrastructure. “Currently there are very few permanent sensors on the ocean floor,” he says. “Scaling the technique would provide thousands of underwater sensors all over the world, which could be revolutionary for seismology and for our understanding of ocean circulation and the impacts of climate change.”
SMART cables
Longer term, environmental engineers are calling for future subsea cables to be fitted with dedicated sensors to measure pressure, temperature and acceleration. This will provide a more comprehensive monitoring system for early detection of seismic events, as well as for climate and ocean observation. The sensing technologies needed for such Science Monitoring And Reliable Telecommunications (SMART) cables have already been demonstrated in underwater geophysical observatories, notably two large-scale networks in Japan that were deployed in the wake of the 2011 Tōhoku earthquake and tsunami. However, more work is needed to demonstrate a practical SMART cable that meets the needs of commercial telecoms operators as well as environmental scientists.
Several pilot projects are now hoping to prove the technology’s feasibility, generally in areas most at risk from seismic events and climate change. As an example, SMART functions are being integrated into a planned communications link between the Pacific islands of New Caledonia and Vanuatu – in the heart of the ‘Ring of Fire’, a horseshoe-shaped string of volcanoes and seismic activity in the Pacific basin – and the five-year programme recently received a $7 million funding boost from the Gordon and Betty Moore Foundation.
Even more ambitious is the CAM-2 project, which in 2025 is due to replace an existing cable that links Portugal with the Atlantic islands of Madeira and the Azores. SMART sensors will be embedded in about 50 repeaters along the 3,700-kilometre route, accounting for about 10% of the €12 million budget. Three tectonic plates converge in the region, so the government-backed project aims to provide early warnings of earthquakes and tsunamis for the Portuguese mainland.
While SMART cables might remain limited to smaller projects for the foreseeable future, Marra hopes that the data they provide might be able to validate and refine the results provided by all-optical methods operating across longer subsea links. “The availability of SMART cables would really help us to improve our technique,” he says. “Combining the rich data from small-scale SMART systems with the reach we can achieve with the existing underwater infrastructure would really benefit the scientific community.”
***
This article has been adapted from an original, Subsea Fiber: Into the deep, published by Optics & Photonics News in March 2023: www.optica-opn.org/link/0323-subsea. With thanks to the editor and publisher.
Our original version of "Undersea information sharing" originally appeared in the print edition of Ingenia 96 (September 2023).
Contributors
Susan Curtis
Author
Keep up-to-date with Ingenia for free
SubscribeRelated content
Electricals & electronics
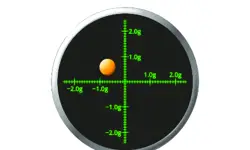
Accelerometers
Used in earthquake measurements, laptops, planes and even in stargazing apps, today’s accelerometers are much smaller than when they were first developed in 1927. Find out how they detect movement and vibration.
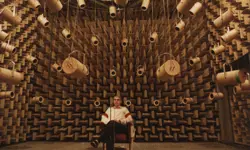
How to maximise loudspeaker quality
Ingenia asked Dr Jack Oclee-Brown, Head of Acoustics at KEF Audio, to outline the considerations that audio engineers need to make when developing high-quality speakers.
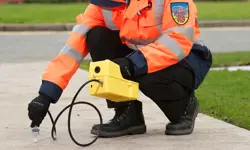
Cable fault locator
The winner of the Institute of Engineering and Technology’s 2014 Innovation Award was EA Technology’s CableSnifferTM, which uses a probe and chemical sensing technology to identify faults, saving energy companies millions of pounds each year.
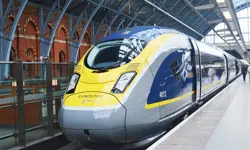
High speed evolution
In December 2010, Eurostar International Ltd awarded a contract for 10 new high speed trains to Siemens. The company has used a system developed over decades to maximise the performance and passenger-carrying ability of its 320km/h trains.
Other content from Ingenia
Quick read
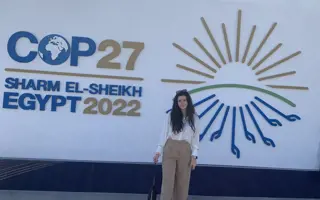
- Environment & sustainability
- Opinion
A young engineer’s perspective on the good, the bad and the ugly of COP27
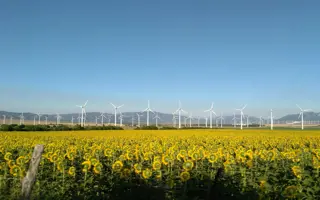
- Environment & sustainability
- Issue 95
How do we pay for net zero technologies?
Quick read
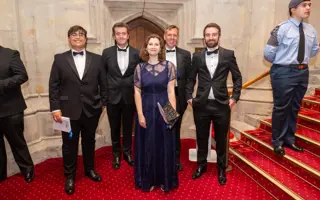
- Transport
- Mechanical
- How I got here
Electrifying trains and STEMAZING outreach
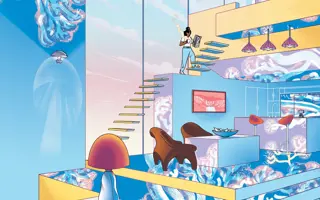
- Civil & structural
- Environment & sustainability
- Issue 95