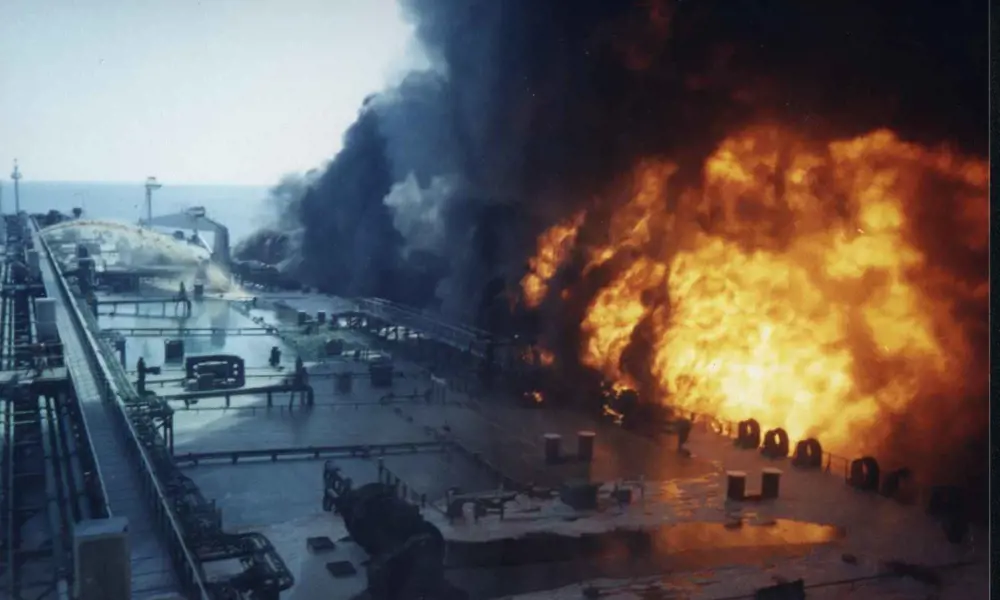
Modelling ships in danger
When a ship runs aground, catches fire, collides with another vessel, begins leaking oil, or suffers another calamity (including terrorism), someone has to decide what to do. That person is usually the ship’s master.
Tankers, bulk carriers, car ferries and cruise liners are large vessels that have complexities to match. Experience counts for much in handling ships, but, when faced with disaster, the ship’s crew needs extra support.
Ships like the Exxon Valdez, Herald of Free Enterprise, Torrey Canyon or, in this decade, Costa Concordia are remembered for the disasters that overtook them. It is with vessels that might otherwise be destined for this notorious category that the Lloyd’s Register Ship Emergency Response Service (SERS) was established. Set up in 1985, SERS is a team of naval experts who can offer advice and make recommendations, providing rapid and continuing contact worldwide from a dedicated response centre onshore.
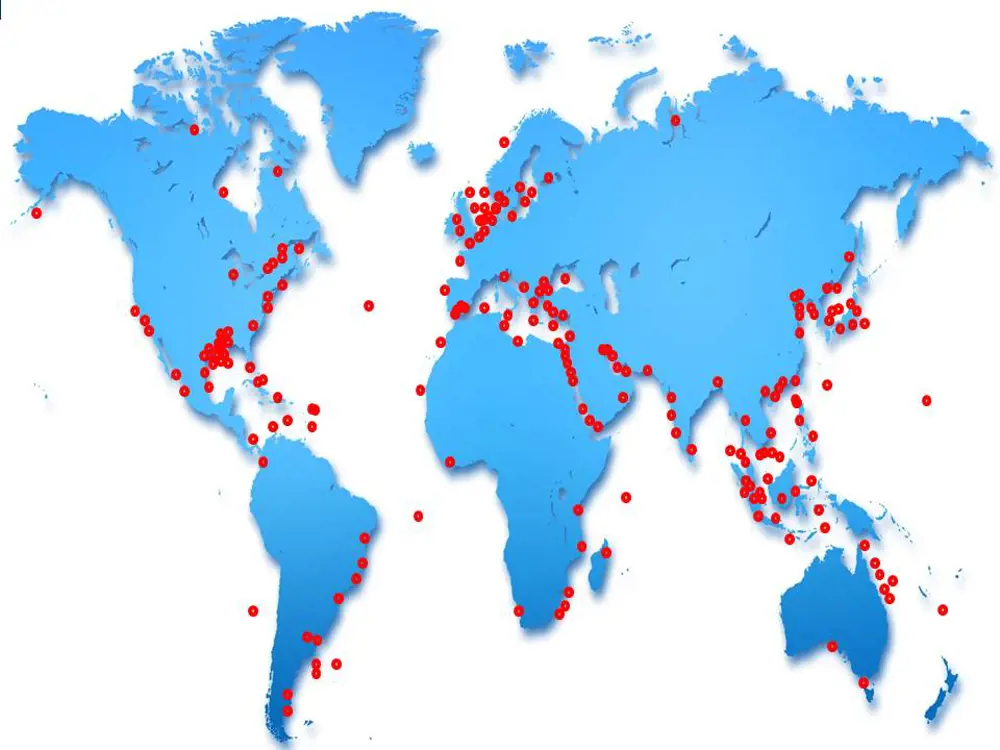
World map showing locations of some of the incidents dealt with by SERS
Knowing what to do
When an incident happens, SERS needs to be alerted as soon as possible. The response teams work in groups of three, comprising a naval architect with expertise in modelling, another naval architect with structural expertise, and a team leader (a third naval architect or a master mariner) responsible for coordinating and driving the chosen strategy and, importantly, liaising with the ship’s master.
The response team will assemble at Lloyd’s Register Marine’s SERS Emergency Response Centre, and establish communication links with the ship and/or client office. Voice, email and fax communication with the ship are usually conducted by INMARSAT or Iridium satellite. Vessel condition and casualty data are then sent to SERS for assessment, the results of which are provided verbally and backed up by written reports. Internet-based web conferencing can be used with clients ashore for interactive assessments, although satellite data speeds currently limit use on board.
The ship’s crew and SERS will both have access to weather, tide and chart data from various providers. SERS is contracted by the ship operator or manager, but will work with third parties such as national, state, port, canal and coastguard authorities, salvors and insurers, as requested or authorised by clients.
The key to providing successful advice lies in the computer modelling of the ship and its cargo. These models can predict what will happen if nothing is done as well as the likely effects of any proposed course of action. SERS computers already hold detailed plans of the structure of each ship subscribing to the service. Software developed over many years can be used to calculate the effects of outside forces bearing on the vessel and, in the light of what is known of its construction, the likelihood of it failing. Stability and floatability can be determined and, as circumstances develop and change, the model can also predict any oil outflow from, and retention within, damaged tanks.
The key to providing successful advice lies in the computer modelling of the ship and its cargo. These models can predict what will happen if nothing is done as well as the likely effects of any proposed course of action
There are 3,200 vessels that are represented in the SERS system. Since its inception, over 5,000 craft have been modelled, representing approximately 150 worker-years of effort. There are ships of all types, ranging from oil tankers, chemical tankers, LPG or LNG gas carriers, container ships and cargo vessels to cruise liners and roll-on-roll-off passenger ferries.
Many factors are at play when a ship gets into serious trouble: the rise and fall of the tide, the local weather and wave action, the location and extent of a grounding point and the measure of any damage to cargo or leakage from fuel oil and ballast tanks. The location and severity of structural damage caused by collision, explosion or fire must also be taken into consideration, as well as the number of flooded compartments of the vessel and whether or not it is listing. Equally influential are the amount, nature and distribution of the cargo; what weight the ship is carrying; if it is liquid, what kind, what quantity and in which tanks; and if in containers, how many and how they are arranged.
Grounding calculation theory
🛳️ How is the grounding reaction force calculated?
By Archimedes’ principle, the weight of a floating object equals the weight of the volume of fluid displaced. The displaced volume of the fluid, and hence the initial weight of the vessel, is calculated using the initial draughts and the corresponding submerged geometry of the ship. The grounded draughts will determine the new displacement.
The difference in the displacements before and after grounding corresponds to the grounding reaction force, taking into account any flooding weights. The change in trim or heel of the vessel will then determine the location of the line of action of this force – so if the reaction force is aft then the vessel will trim forward (or if to port will heel to starboard). The diagram illustrates how the grounding reaction force is calculated in this way.
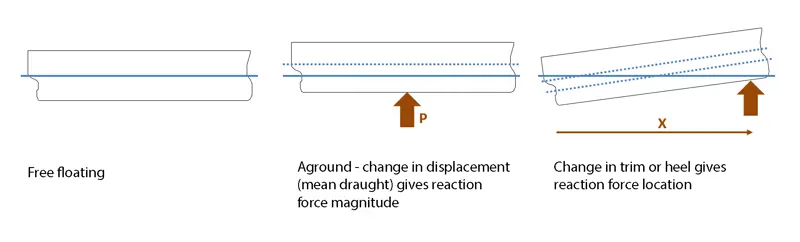
Information needed
SERS assessment procedure follows three established steps: confirmation of its model of the ship condition before the casualty, evaluation of the casualty condition, and consideration of remedial action.
The initial condition of the ship requires a full description of the loading of the vessel. Onboard, the condition status is maintained by the ship’s loading records. Tank levels are generally monitored by gauges that input to the loading computer, but small tanks may be sounded manually and weights then input to the assessment data. Once all weights have been input and the reported draughts agree with SERS calculations, confirmation of the facts known is requested. Confirmation is important as all calculations that follow are based on these data. Then, the vessel’s casualty condition can be modelled – see Grounding calculation theory.
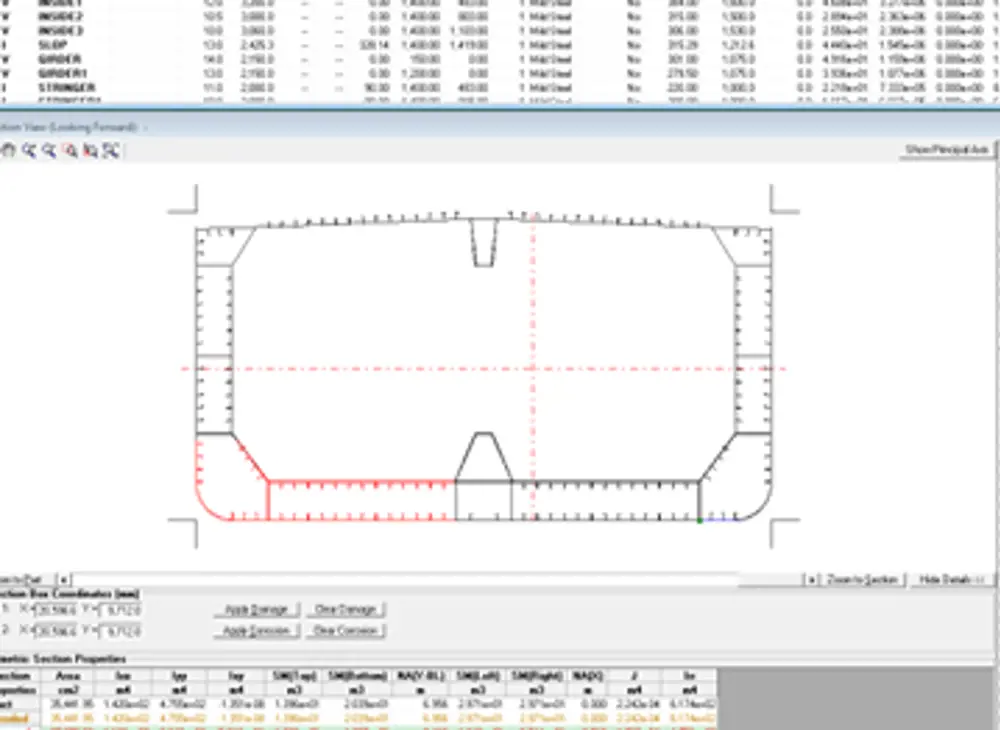
2D section modelling is used to calculate damage residual longitudinal strength (beam strength). Modelling tools represent the structural elements of plates and stiffeners and calculate the geometric section properties. Regions of the cross section that are considered to be ineffective are then removed from the model. Remaining vertical bending moment and shear force strength can be calculated, either with respect to design stresses, with a margin against yield, or for ultimate strength, allowing some buckling or yield
Courses of action
While the computer can calculate a vessel’s stability, residual strength and resistance to stress, it cannot by itself provide a course of remedial action. Nor is there necessarily only one course of action that could be taken. The SERS team leader has to exercise judgement before advising the crew on what to do with their stricken vessel.
Casualty situations can be complex. SERS technical assessment procedure uses its own nautical aide memoire: ‘SS FOG and Tide’. This assesses:
- Strength – considering the hull girder as a ‘beam’ under loadings of weight, buoyancy and wave forces
- Stability – the capacity to resist overturning effects of wind, waves, weight and movement of liquid cargo
- Floatability – reserve of buoyancy, freeboard and heel/trim
- Outflow – loss of liquid cargo
- Grounding – reaction force, its distribution and location; and refloating of ship
- Tide – which may greatly influence the above and should not be forgotten, such as when aiming to refloat at high water, consider also loads on the ship at low water
Primary assessments are supported by a variety of tools, such as 2D section property modelling of damage residual strength. The range of what might be recommended is broad. It might be to do nothing if the next high tide can be counted on to refloat a grounded vessel. It could be to pump out water ballast or offload part of the cargo. If a tanker is holed near the water line on one side, it might make sense to re-ballast it in such a way as to produce an artificial list to raise damage above the waterline in order to retain cargo and/or allow the compartment to be pumped out.
The aim throughout is to get the vessel back into normal service as rapidly as possible while at the same time limiting damage to the environment, particularly from leaking oil
In the case of grounding in rough seas, a vessel could be battered against rocks or subject to shearing forces from wave action. The first step could be to pump more water into its ballast tanks and so induce it to settle more firmly. This may seem a contrary course of action, but a vessel that is securely grounded and moving less in response to wave action may ultimately fare better than one still bobbing around. Once the storm has abated, the ship’s ballast tanks can be emptied and attempts made to refloat it. Liquid cargo may need to be pumped to adjacent tanks to stabilise a vessel, or alter its orientation in some way – see Running aground.
The team will also advise on the most cost-effective temporary repair arrangements, and the safest route to a harbour where permanent repairs can be effected. The aim throughout is to get the vessel back into normal service as rapidly as possible while at the same time limiting damage to the environment, particularly from leaking oil. However, the final decision on what to do rests with the ship’s master, although SERS recommendations are seldom disregarded.
Running aground
🖥️ The complexity in modelling tanker grounding
In a typical scenario for tanker grounding, where the vessel has run aground on a rocky pinnacle, which has torn open the bottom of the ship flooding the double-hull (ballast tanks) and the inner bottom allowing some cargo to escape. The graphical interface is used to manipulate the 3D computer model of the vessel. Loadings have already been applied to generate the initial condition model and it has been checked against the reported condition. Now the grounding casualty has been modelled revealing that there is:
1. Flooding - the water ballast tank No.1 Port is open to sea and has flooded with seawater
2. Outflow - cargo tank 1 Port, above, is damaged at the hole marked. The cargo has escaped until there is hydrostatic equilibrium. (Note also the oil-water interface in tank to level of the hole, illustrating how water ingress is also tracked)
3. Grounding - After setting the attitude of the vessel (draughts, heel and trim), and by taking into account flooding of the ballast tank and equilibration of the cargo then the computer will calculate the grounding force at the location shown.
4. Strength capacity - Hull girder bending moment limits (blue): maximum (‘hogging’) and minimum (‘sagging’). The curve is adjusted to take account of grounding damage (dotted line)
5. Strength assessment - The actual still water bending moment is outside (below) the limits. This is due to upward grounding force forward. Note that in this case the greatest exceedence of the limit is midship, where the hull girder is intact and away from the weakened damage area at the grounding point.
This example illustrates the potential complexity of these scenarios. Suppose the vessel is to be re-floated by taking actions to raise the hull clear of the rock. This would normally be timed to take advantage of high water and carried out by movement of cargo internally or by offload, or ballasting/de-ballasting. However, strength and stability must also be controlled during the refloating and for the free-floating condition. Meanwhile pollution must be minimised. The vessel may also need to go through a low tide before the high water or if refloating is unsuccessful other options may be needed.
The team will also advise on the most cost-effective temporary repair arrangements, and the safest route to a harbour where permanent repairs can be effected. The aim throughout is to get the vessel back into normal service as rapidly as possible while at the same time limiting damage to the environment, particularly from leaking oil. However, the final decision on what to do rests with the ship’s master, although SERS recommendations are seldom disregarded.
Maritime Maisie
One year ago, SERS had to deal with a catastrophic shipping failure on the southeastern tip of the Korean peninsula. In December 2013, near the South Korean city of Busan, the tanker Maritime Maisie, carrying a 44,000-tonne cargo of chemicals, collided with another vessel, the Gravity Highway. The latter suffered some damage to its bow, but not to the same extent as Maritime Maisie, which had a large hole in its port side. The cargo caught fire and burned for a few hours in a pool on the sea, and uncontrollably for nearly a month inside the damaged tanks. This distorted the steel plates and girders of the ship and left it structurally weakened: a development made all the more threatening by periodically turbulent weather and waves up to 4m in height.
Although SERS was contacted immediately for advice on what to do, its efforts were initially hampered by the lack of information. The severity of the fire had forced the crew to abandon ship, and the only available sources of information about its condition were media photographs and some video footage, plus what could be inferred from knowledge of the state of the vessel with which it had collided. The SERS team modelled Maritime Maisie’s predicament as best it could, made an estimate of the likely damage, and determined that the vessel was not in immediate danger of breaking up or sinking. The ship did, however, drift into Japanese waters until tugs were brought in to prevent its further movement. Once the fire was extinguished and the ship had cooled down, surveyors were able to assess the vessel from a launch, and later to board it. This revealed that the original SERS damage assessments had been accurate. But, by this time the fire had almost completely destroyed Maritime Maisie’s deck, and the vessel was being held together only by its starboard side, centre-line longitudinal bulkhead and bottom. This further weakening posed a real threat to its structural integrity, even to the extent that it might break in two. Further computer modelling by SERS suggested that if this did happen, both halves would in fact continue to float – see Maritime Maisie modelling diagrams. In the event, a breakage did not occur, and the vessel was declared safe to tow to a port.
Although SERS was contacted immediately for advice on what to do, its efforts were initially hampered by the lack of information
Mindful of the possibly hazardous nature of Maritime Maisie’s cargo, and of the vessel’s weakened condition, both Korea and Japan initially refused to authorise a safe haven. In the light of this impasse, the tugs securing the vessel at sea were required for almost 100 days. Eventually, the SERS assessment of the vessel’s stability and integrity was accepted, and Maritime Maisie berthed in the South Korean port of Ulsan for its chemical cargo to be removed to another vessel moored alongside it. To ensure that the removal of the cargo would not add to the stress imposed on the already weakened structure, SERS devised a nine-stage process for systematically pumping the cargo, tank by tank, into the second vessel, and reballasting. Only then were surveyors able to assess the full extent of the damage Maritime Maisie had suffered. The outcome of the incident could have been far worse, and a large loss of heavy fuel oil had been avoided. The vessel is now being repaired and it is hoped that it soon will return to service.
Refloating
🛢️ SERS reacting to a carrier with 800 tonnes of oil going aground
In 2001, the Nol Schedar, an 80,000-tonne bulk carrier, went aground fully laden with coal in fair weather on a reef in the Philippines. it was also carrying 800 tonnes of heavy fuel oil, diesel oil and lubricating oil. Double bottom fuel oil tanks were breached, but outflow was negated as the less dense oil floated on the seawater driven in by the higher external pressure. The inner bottom was not breached, but SERS had to act quickly to prevent the situation getting worse.
The SERS team ascertained that the grounding point was toward the bows, and not in the more threatening mid region of the ship. The centre of reaction was established around which any bending of the hull might take place, and the consequent forces on its structure were determined. From this, they were able to calculate how much of the vessel’s cargo needed to be offloaded to refloat it. They also suggested the sequence in which it should be taken: important because, in these circumstances, far from relieving the strain on a hull, a poorly judged removal of cargo could increase it.
At this point, nature chose to reassert itself. The ocean swell rose to 6m, the ship started to move and began to be pounded on the reef. The consequent damage led to the flooding of six of its water ballast tanks. To limit further movement of the ship, and so minimise the risk of even greater damage, it was decided to add more ballast. This altered the positioning of the ship in relation to the reef. A new set of calculations of the stress on the hull was required, and this in turn dictated an alteration to the optimum sequence of the subsequent cargo removal. The dynamic nature of most marine accidents means that advice often has to be modified as events unfold.
The offloading of the cargo proceeded according to the salvor’s plan, validated by SERS and, with the help of tugs, the vessel floated free. At this point, however, the sea was breaking over the vessel’s decks and there was further unexpected flooding. SERS modelled the effects and confirmed the floating attitude and reserve of buoyancy. The authorities at the port where the ship’s owners hoped to make temporary repairs voiced concern about its safe entry. They feared the vessel might block the entrance to a local power station.
SERS revisited the ballasting calculations. They were able to convince the port authorities that once a further 2,000 tonnes of the ship’s cargo had been offloaded, it could be docked in safety. Finally, following the removal of more of its cargo, the ship was allowed to proceed for temporary repairs, and then to another shipyard for permanent repairs. The Nol Schedar is still in service today.
Risk of an accident
Although the quantity of world shipping increases year by year and so too does the number of ships in SERS, the number of incidents that SERS deals with remains relatively steady at between 25 and 30 annually. This suggests that the accident rate is dropping, a consequence of improvements in operational and navigational safety and tighter regulation. Environmental pollution by oil in particular has galvanised changes in the last of these.
This suggests that the accident rate is dropping, a consequence of improvements in operational and navigational safety and tighter regulation
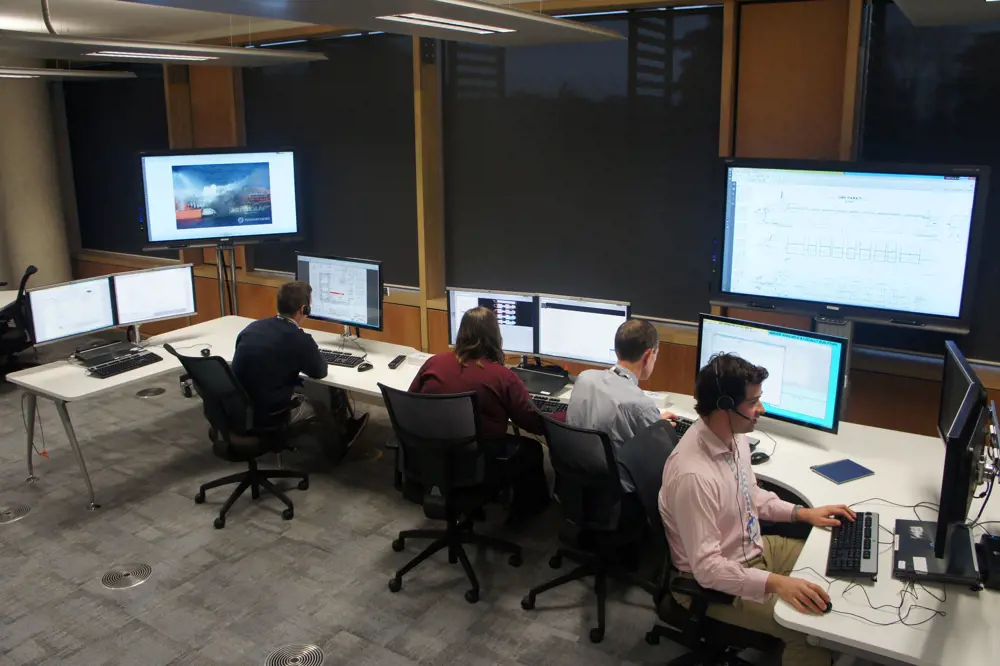
Following the 1989 Exxon Valdez incident, the US Oil Pollution Act (1990) was drawn up, requiring that oil tankers visiting the US should have prompt access to “computerised, shore-based damage stability and residual structural strength calculation programs”. The vision that had led to the creation of SERS some years before was now realised in legislation.
In January 2007, the International Maritime Organisation introduced an annex to its International Convention for the Prevention of Pollution from Ships which extended rules to oil tankers of 5,000 DWT (deadweight tonnage) and above, worldwide. More recently, US non-tank vessel (NTV) legislation has addressed associated pollution risk from vessel bunker oils, by encouraging, but not mandating enrolment in such response services.
The average cost of an accident to a ship owner is around $2.2 million – although the figure can turn out to be several times greater. The creation of SERS was a response to the concerns of tanker owners facing the risks of transporting oil in ever-larger quantities. Now, with 550 clients and more than 3,200 vessels of all kinds, SERS is still the leading single provider among the emergency response organisations.
Since 1985, SERS has provided nearly 40,000 ship-years of cover, responding to 390 emergencies and conducting 1,300 exercises.
It offers regular training courses to clients, who are also provided with an emergency manual that includes a casualty report form detailing all the information that shore-based advisers will need in the event of an accident. Such training is essential. Owners or operators can bring senior mariners to their own offices, wherever in the world these are located, and then rehearse a fictional damage scenario in which the SERS team in Southampton acts exactly as it would in the circumstances of an actual incident. Captains who later need SERS advice for real will not only know in principle how the system works, but have a feel of what it is like to use it. SERS staff too can use these simulations for their own training purposes, ensuring that each team’s performance is kept up to the mark.
SERS has been an entry point to the profession for many young naval architects
In November 2014, SERS commenced the next stage of its development: a move into the new Global Technology Centre, a purpose-built building within Southampton University campus. Here they became part of a 400-strong contingent of Lloyd’s Register staff, all concerned in one way or another with the future of shipping and the services that underpin it. Southampton University has an international reputation in naval architecture, fluid dynamics and acoustics, and oceanography. This move will bring SERS close to researchers responsible for developing new technology, a proximity that will encourage its continued introduction into routine service. SERS has been an entry point to the profession for many young naval architects.
Much has been learned from the operation of emergency response services. Most SERS incidents have operational causes such as manoeuvring or navigational error, or incorrect navigational aids resulting in collision or grounding. Equipment failures are relatively few. Sometimes blackouts or steering gear failure will result in collision or grounding, but improvements in design specifications over the years have mitigated casualty consequences such as double-hulls and damage stability standards.
The development of design and operational standards has followed extensive efforts from both national and international regulatory bodies. SERS has contributed to the development of industry standards for emergency response services, such as those recently published by The Oil Companies International Marine Forum (OCIMF) that generally confirm the arrangements envisaged by SERS back in the 1980s.
So, if two vessels were to collide in the Channel or a bulk carrier ran aground somewhere, there is a chance that the Southampton office will be involved. No matter what time the incident happens, there will be SERS staff checking the registered ship’s plans, local tide patterns and offering support within the hour.
***
The authors would like to thank Geoff Watts, science and medical writer and broadcaster, for his help in writing this article.
This article has been adapted from "Modelling ships in danger", which originally appeared in the print edition of Ingenia 61 (December 2014).
Contributors
David Prentice, Lead Naval Architect for SERS, is responsible for maintenance and development of its systems. David trained at Lloyd’s Register as a ship surveyor and has been a SERS emergency response team leader for 15 years leading on responses to nearly 200 emergencies and 550 exercises.
Wijendra Peiris, Manager at SERS, is responsible for its technical leadership and provision of technical expertise and advice to clients. He has been a SERS emergency response leader since 2009 and involved with leading responses in over 100 emergencies and exercises.
Nick Brown, Marine Communications Manager, is responsible for promoting and protecting the interests of Lloyd’s Register’s Marine business. He has led crisis training courses world-wide and, with Brent Pyburn, was co-author of INTERTANKO’s Guiding Principles to Emergency Management and Crisis Communications.
Keep up-to-date with Ingenia for free
SubscribeRelated content
Maritime & naval
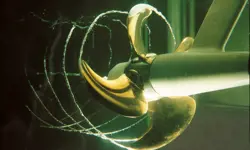
Quieter, more efficient propellers
Göran Grunditz, Manager of the Rolls-Royce Hydrodynamics Research Centre, explains the challenges when reducing noise from marine propellers for passengers onboard ships and for military vessels that seek to avoid detection, while maintaining a high thrust per input power and maximising efficiency.
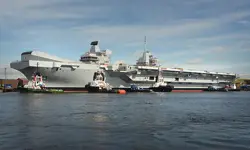
Building Britain's biggest warships
The 1998 Strategic Defence Review called for the UK Armed Forces to be able to operate with more agility to confront situations on a worldwide basis and resulted in the requirement for two new Queen Elizabeth Class aircraft carriers, HMS Queen Elizabeth and HMS Prince of Wales, one of the UK's largest engineering projects.
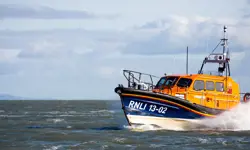
Against the tide
The Royal National Lifeboat Institution introduced the Shannon class all-weather lifeboat in 2014, which uses cutting-edge technology, is faster and more manoeuvrable than existing vessels, and has a quicker and safer launch and recovery system. Read about the design and production of the lifeboat.
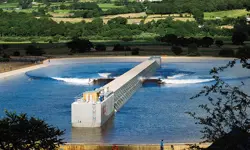
How to create the perfect wave
From small waves lapping at your feet and swells suitable for surfing to storm waves for testing structures and even tsunamis, waves of any shape and any size can now be engineered. What are the techniques and conditions needed to model waves and what makes some more powerful than others?
Other content from Ingenia
Quick read
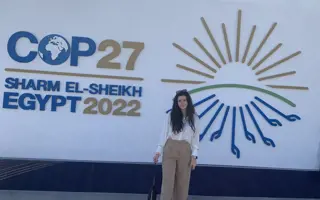
- Environment & sustainability
- Opinion
A young engineer’s perspective on the good, the bad and the ugly of COP27
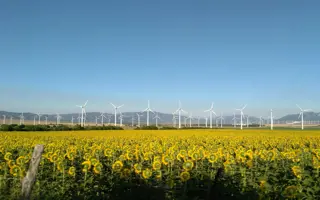
- Environment & sustainability
- Issue 95
How do we pay for net zero technologies?
Quick read
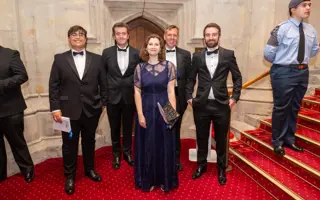
- Transport
- Mechanical
- How I got here
Electrifying trains and STEMAZING outreach
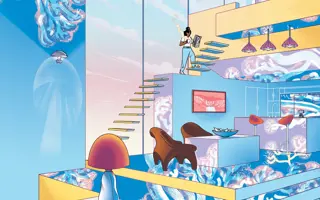
- Civil & structural
- Environment & sustainability
- Issue 95